Abstract
Here we present our progress in inducing an ectopic brown adipose tissue (BAT) phenotype in skeletal muscle (SKM) as a potential gene therapy for obesity and its comorbidities. We used ultrasound-targeted microbubble destruction (UTMD), a novel targeted, non-viral approach to gene therapy, to deliver genes in the BAT differentiation pathway into rodent SKM to engineer a thermogenic BAT phenotype with ectopic mUCP-1 overexpression. In parallel, we performed a second protocol using wild-type Ucp-1-null knockout mice to test whether the effects of the gene therapy are UCP-1 dependent. Our main findings were a robust cellular presence of mUCP-1 immunostaining (IHC), significantly higher expression levels of mUCP-1 measured by qRT-PCR, and highest temperature elevation measured by infrared thermography in the treated thigh, achieved in rats after delivering the UTMD-PRDM16/PGC-1a/BMP7/hyPB gene cocktail. Interestingly, the weight loss obtained in the treated rats with the triple gene delivery, never recovered the levels observed in the controls in spite of food intake recovery. Our results establish the feasibility of minimally invasive UTMD gene-based therapy administration in SKM, to induce overexpression of ectopic mUCP-1 after delivery of the thermogenic BAT gene program, and describe systemic effects of this intervention on food intake, weight loss, and thermogenesis.
This is a preview of subscription content, access via your institution
Access options
Subscribe to this journal
Receive 12 print issues and online access
$259.00 per year
only $21.58 per issue
Buy this article
- Purchase on Springer Link
- Instant access to full article PDF
Prices may be subject to local taxes which are calculated during checkout





Similar content being viewed by others
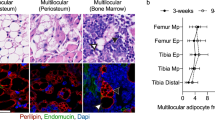
References
Ng M, Fleming T, Robinson M, Thomson B, Graetz N, Margono C, et al. Global, regional, and national prevalence of overweight and obesity in children and adults during 1980-2013: a systematic analysis for the Global Burden of Disease Study 2013. Lancet (Lond, Engl). 2014;384:766–81.
Aslibekyan S, Garvey WT. Obesity: obesity and cardiometabolic disease - more than meets the eye. Nat Rev Endocrinol. 2017;13:566–8.
Ochner CN, Barrios DM, Lee CD, Pi-Sunyer FX. Biological mechanisms that promote weight regain following weight loss in obese humans. Physiol Behav. 2013;120:106–13.
Lagerros YT, Rossner S. Obesity management: what brings success? Ther Adv Gastroenterol. 2013;6:77–88.
Betz MJ, Enerback S. Targeting thermogenesis in brown fat and muscle to treat obesity and metabolic disease. Nat Rev Endocrinol. 2017;14:77–87.
Halpern B, Mancini MC, Halpern A. Brown adipose tissue: what have we learned since its recent identification in human adults. Arq Bras Endocrinol Metabol. 2014;58:889–99.
Spiegelman BM. Banting Lecture 2012: regulation of adipogenesis: toward new therapeutics for metabolic disease. Diabetes. 2013;62:1774–82.
Katterle Y, Keipert S, Hof J, Klaus S. Dissociation of obesity and insulin resistance in transgenic mice with skeletal muscle expression of uncoupling protein 1. Physiol Genom. 2008;32:352–9.
Voigt A, Katterle Y, Kahle M, Kluge R, Schurmann A, Joost HG, et al. Skeletal muscle mitochondrial uncoupling prevents diabetes but not obesity in NZO mice, a model for polygenic diabesity. Genes Nutr. 2015;10:57.
Keipert S, Voigt A, Klaus S. Dietary effects on body composition, glucose metabolism, and longevity are modulated by skeletal muscle mitochondrial uncoupling in mice. Aging Cell. 2011;10:122–36.
O’Neill SM, Hinkle C, Chen SJ, Sandhu A, Hovhannisyan R, Stephan S, et al. Targeting adipose tissue via systemic gene therapy. Gene Ther. 2014;21:653–61.
Lukashev AN, Zamyatnin AA Jr.. Viral vectors for gene therapy: current state and clinical perspectives. Biochem (Mosc). 2016;81:700–8.
Thomas CE, Ehrhardt A, Kay MA. Progress and problems with the use of viral vectors for gene therapy. Nat Rev Genet. 2003;4:346–58.
Chen ZY, Yang F, Lin Y, Zhang JS, Qiu RX, Jiang L, et al. New development and application of ultrasound targeted microbubble destruction in gene therapy and drug delivery. Curr Gene Ther. 2013;13:250–74.
Geis NA, Katus HA, Bekeredjian R. Microbubbles as a vehicle for gene and drug delivery: current clinical implications and future perspectives. Curr Pharm Des. 2012;18:2166–83.
Zhang L, Sun Z, Ren P, Lee RJ, Xiang G, Lv Q, et al. Ultrasound-targeted microbubble destruction (UTMD) assisted delivery of shRNA against PHD2 into H9C2 cells. PLoS ONE. 2015;10:e0134629.
Chen S, Shimoda M, Chen J, Matsumoto S, Grayburn PA. Transient overexpression of cyclin D2/CDK4/GLP1 genes induces proliferation and differentiation of adult pancreatic progenitors and mediates islet regeneration. Cell Cycle (Georget, Tex). 2012;11:695–705.
Frost PA, Chen S, Mezzles MJ, Voruganti VS, Nava-Gonzalez EJ, Arriaga-Cazares HE, et al. Successful pharmaceutical-grade streptozotocin (STZ)-induced hyperglycemia in a conscious tethered baboon (Papio hamadryas) model. J Med Primatol. 2015;44:202–17.
Chen S, Bastarrachea RA, Roberts BJ, Voruganti VS, Frost PA, Nava-Gonzalez EJ, et al. Successful beta cells islet regeneration in streptozotocin-induced diabetic baboons using ultrasound-targeted microbubble gene therapy with cyclinD2/CDK4/GLP1. Cell Cycle (Georget, Tex). 2014;13:1145–51.
Vu V, Liu Y, Sen S, Xu A, Sweeney G. Delivery of adiponectin gene to skeletal muscle using ultrasound targeted microbubbles improves insulin sensitivity and whole body glucose homeostasis. Am J Physiol Endocrinol Metab. 2013;304:E168–75.
Farmer SR. Brown fat and skeletal muscle: unlikely cousins? Cell. 2008;134:726–7.
Bastarrachea RA, Chen J, Kent JW Jr., Nava-Gonzalez EJ, Rodriguez-Ayala E, Daadi MM, et al. Engineering brown fat into skeletal muscle using ultrasound-targeted microbubble destruction gene delivery in obese Zucker rats: proof of concept design. IUBMB Life. 2017;69:745–55.
Crane JD, Mottillo EP, Farncombe TH, Morrison KM, Steinberg GR. A standardized infrared imaging technique that specifically detects UCP1-mediated thermogenesis in vivo. Mol Metab. 2014;3:490–4.
Jang C, Jalapu S, Thuzar M, Law PW, Jeavons S, Barclay JL, et al. Infrared thermography in the detection of brown adipose tissue in humans. Physiol Rep. 2014;2:e12167.
Strauss JA, Shaw CS, Bradley H, Wilson OJ, Dorval T, Pilling J, et al. Immunofluorescence microscopy of SNAP23 in human skeletal muscle reveals colocalization with plasma membrane, lipid droplets, and mitochondria. Physiol Rep. 2016;4:e12662.
Kimmel AR, Sztalryd C. Perilipin 5, a lipid droplet protein adapted to mitochondrial energy utilization. Curr Opin Lipidol. 2014;25:110–7.
Frontini A, Cinti S. Distribution and development of brown adipocytes in the murine and human adipose organ. Cell Metab. 2010;11:253–6.
Cinti S. Between brown and white: novel aspects of adipocyte differentiation. Ann Med. 2011;43:104–15.
Cui XB, Chen SY. White adipose tissue browning and obesity. J Biomed Res. 2016;31:1–2.
Christian M, Parker MG. The engineering of brown fat. J Mol Cell Biol. 2010 Feb;2:23–5.
Nicholls DG. The physiological regulation of uncoupling proteins. Biochim Biophys Acta. 2006;1757:459–66.
Tang W, Zeve D, Suh JM, Bosnakovski D, Kyba M, Hammer RE, et al. White fat progenitor cells reside in the adipose vasculature. Science. 2008;322:583–6.
Atit R, Sgaier SK, Mohamed OA, Taketo MM, Dufort D, Joyner AL, et al. Beta-catenin activation is necessary and sufficient to specify the dorsal dermal fate in the mouse. Dev Biol. 2006;296:164–76.
Timmons JA, Wennmalm K, Larsson O, Walden TB, Lassmann T, Petrovic N, et al. Myogenic gene expression signature establishes that brown and white adipocytes originate from distinct cell lineages. Proc Natl Acad Sci USA. 2007;104:4401–6.
Mezentseva NV, Kumaratilake JS, Newman SA. The brown adipocyte differentiation pathway in birds: an evolutionary road not taken. BMC Biol. 2008;6:17.
Rodriguez A, Becerril S, Ezquerro S, Mendez-Gimenez L, Fruhbeck G. Crosstalk between adipokines and myokines in fat browning. Acta Physiol (Oxf). 2017;219:362–81.
Li F, Li Y, Duan Y, Hu CA, Tang Y, Yin Y. Myokines and adipokines: involvement in the crosstalk between skeletal muscle and adipose tissue. Cytokine Growth Factor Rev. 2017;33:73–82.
Bach AD, Arkudas A, Tjiawi J, Polykandriotis E, Kneser U, Horch RE, et al. A new approach to tissue engineering of vascularized skeletal muscle. J Cell Mol Med. 2006;10:716–26.
Keipert S, Ost M, Johann K, Imber F, Jastroch M, van Schothorst EM, et al. Skeletal muscle mitochondrial uncoupling drives endocrine cross-talk through the induction of FGF21 as a myokine. Am J Physiol Endocrinol Metab. 2014;306:E469–82.
Fisher FM, Kleiner S, Douris N, Fox EC, Mepani RJ, Verdeguer F, et al. FGF21 regulates PGC-1alpha and browning of white adipose tissues in adaptive thermogenesis. Genes Dev. 2012;26:271–81.
Matos LL, Trufelli DC, de Matos MG, da Silva Pinhal MA. Immunohistochemistry as an important tool in biomarkers detection and clinical practice. Biomark Insights. 2010;5:9–20.
Gjerdrum LM, Sorensen BS, Kjeldsen E, Sorensen FB, Nexo E, Hamilton-Dutoit S. Real-time quantitative PCR of microdissected paraffin-embedded breast carcinoma: an alternative method for HER-2/neu analysis. J Mol Diagn. 2004;6:42–51.
Nistor A, Watson PH, Pettigrew N, Tabiti K, Dawson A, Myal Y. Real-time PCR complements immunohistochemistry in the determination of HER-2/neu status in breast cancer. BMC Clin Pathol. 2006;6:2.
Sawada T, Miyoshi H, Shimada K, Suzuki A, Okamatsu-Ogura Y, Perfield JW, et al. Perilipin overexpression in white adipose tissue induces a brown fat-like phenotype. PLoS ONE. 2010;5:e14006.
Tseng YH, Kokkotou E, Schulz TJ, Huang TL, Winnay JN, Taniguchi CM, et al. New role of bone morphogenetic protein 7 in brown adipogenesis and energy expenditure. Nature. 2008;454:1000–4.
Kajimura S, Seale P, Kubota K, Lunsford E, Frangioni JV, Gygi SP, et al. Initiation of myoblast to brown fat switch by a PRDM16-C/EBP-beta transcriptional complex. Nature. 2009;460:1154–8.
Ventura-Clapier R, Garnier A, Veksler V. Transcriptional control of mitochondrial biogenesis: the central role of PGC-1alpha. Cardiovasc Res. 2008;79:208–17.
Seale P. Transcriptional regulatory circuits controlling brown fat development and activation. Diabetes. 2015;64:2369–75.
Seale P, Kajimura S, Yang W, Chin S, Rohas LM, Uldry M, et al. Transcriptional control of brown fat determination by PRDM16. Cell Metab. 2007;6:38–54.
Hill JO, Peters JC, Wyatt HR. Using the energy gap to address obesity: a commentary. J Am Diet Assoc. 2009;109:1848–53.
Melby CL, Paris HL, Foright RM, Peth J. Attenuating the biologic drive for weight regain following weight loss: must what goes down always go back up? Nutrients. 2017;9:468.
Schutz Y, Byrne NM, Dulloo A, Hills AP. Energy gap in the aetiology of body weight gain and obesity: a challenging concept with a complex evaluation and pitfalls. Obes Facts. 2014;7:15–25.
Dulloo AG. Explaining the failures of obesity therapy: willpower attenuation, target miscalculation or metabolic compensation? Int J Obes. 2012;36:1418–20. 2005
Dulloo AG. Translational issues in targeting brown adipose tissue thermogenesis for human obesity management. Ann N Y Acad Sci. 2013;1302:1–10.
Major GC, Doucet E, Trayhurn P, Astrup A, Tremblay A. Clinical significance of adaptive thermogenesis. Int J Obes. 2007;31:204–12. 2005
Marlatt KL, Ravussin E. Brown adipose tissue: an update on recent findings. Curr Obes Rep. 2017;6:389–96.
Park A, Kim WK, Bae KH. Distinction of white, beige and brown adipocytes derived from mesenchymal stem cells. World J Stem Cells. 2014;6:33–42.
Wood WM, Etemad S, Yamamoto M, Goldhamer DJ. MyoD-expressing progenitors are essential for skeletal myogenesis and satellite cell development. Dev Biol. 2013;384:114–27.
Zhou Q, Brown J, Kanarek A, Rajagopal J, Melton DA. In vivo reprogramming of adult pancreatic exocrine cells to beta-cells. Nature. 2008;455:627–32.
Chen S, Shohet RV, Bekeredjian R, Frenkel P, Grayburn PA. Optimization of ultrasound parameters for cardiac gene delivery of adenoviral or plasmid deoxyribonucleic acid by ultrasound-targeted microbubble destruction. J Am Coll Cardiol. 2003;42:301–8.
Cary LC, Goebel M, Corsaro BG, Wang HG, Rosen E, Fraser MJ. Transposon mutagenesis of baculoviruses: analysis of Trichoplusia ni transposon IFP2 insertions within the FP-locus of nuclear polyhedrosis viruses. Virology. 1989;172:156–69.
Yusa K, Zhou L, Li MA, Bradley A, Craig NL. A hyperactive piggyBac transposase for mammalian applications. Proc Natl Acad Sci USA. 2011;108:1531–6.
Author contributions
All authors listed, have made substantial, direct, and intellectual contribution to the work, and approved it for publication. In particular: RAB conceived the rationale to use UTMD in muscle tissue to express BAT thermogenic genes. SC and RAB designed the experiment. SC, J-SS, and PH performed the experiments, contributed reagents/materials/analysis tools and designed the figures. RAB, EJN-G, and JWK wrote the introduction and analyzed the data; RAB, ER-A, and AL-N wrote the conclusions and performed literature review. JWK, RADF, and PAG are senior authors that proof-read the manuscript and substantially contributed to the conclusions and study design.
Author information
Authors and Affiliations
Corresponding author
Ethics declarations
Conflict of interest
The authors declare that they have no conflict of interest.
Rights and permissions
About this article
Cite this article
Chen, S., Bastarrachea, R.A., Shen, JS. et al. Ectopic BAT mUCP-1 overexpression in SKM by delivering a BMP7/PRDM16/PGC-1a gene cocktail or single PRMD16 using non-viral UTMD gene therapy. Gene Ther 25, 497–509 (2018). https://doi.org/10.1038/s41434-018-0036-5
Received:
Revised:
Accepted:
Published:
Issue Date:
DOI: https://doi.org/10.1038/s41434-018-0036-5