- 1Department of Neurosurgery, The Affiliated Hospital of Qingdao University, Qingdao, China
- 2Department of Emergency Surgery, The Affiliated Hospital of Qingdao University, Qingdao, China
- 3Department of Radiology, the Affiliated Hospital of Qingdao University, Qingdao, China
- 4Department of Outpatient, The Affiliated Hospital of Qingdao University, Qingdao, China
CD24 has emerged as a molecule of significant interest beyond the oncological arena. Recent studies have unveiled its surprising and diverse roles in various biological processes and diseases. This review encapsulates the expanding spectrum of CD24 functions, delving into its involvement in immune regulation, cancer immune microenvironment, and its potential as a therapeutic target in autoimmune diseases and beyond. The ‘magic’ of CD24, once solely attributed to cancer, now inspires a new paradigm in understanding its multifunctionality in human health and disease, offering exciting prospects for medical advancements.
Introduction
CD24 is a glycosylphosphatidylinositol-anchored protein that was first identified in 1978 as a B-cell differentiation antigen (1). It is expressed in a wide range of tissues and cell types, including hematopoietic stem cells, B and T lymphocytes, epithelial cells, and neural cells (2–11). CD24 has been found to play a role in a variety of physiological and pathological processes, including cell adhesion, migration, differentiation, and apoptosis (12).
In recent years, there has been growing interest in phagocytosis checkpoints, especially CD24, as potential therapeutic targets for cancer treatment (13–15) (see Figure 1). Given the heterogeneity of in post-translational modifications, CD24 has been implicated in tumor growth, invasion, and metastasis, and has been suggested as a potential marker for cancer prognosis and therapy (14, 16–21). More importantly, growing research has uncovered vital functions of CD24 in a range of pathological states, such as autoimmune disorders (22–24), sepsis (25) metabolic disorders (26), graft vs host diseases (27).
In this review, we offer an in-depth examination of CD24, encompassing fundamental principles and pertinent pathways. We also emphasize the significance of CD24 in both cancer and non-neoplastic conditions. Furthermore, we shed light on the ongoing clinical progress in targeting CD24 and identify the obstacles and possible remedies within the realm of cancer immunotherapy and non-neoplastic disorders. Our objective is to not only advance our comprehension of the existing CD24 research landscape but also to delve into the prospects of CD24-based immunotherapeutic approaches.
Structure of CD24
CD24 is expressed on various cell types, including immune cells, neural cells, and cancer cells, and it is a glycosyl-phosphatidylinositol (GPI)-anchored protein with distinct domains: an intracellular domain, a transmembrane domain, and a heavily glycosylated extracellular domain (2–11, 28, 29). The extracellular domain of CD24 has varying numbers of N-linked and O-linked glycosylation points, playing a role in controlling CD24-driven cell attachment and signal transmission (30). It has one N-glycosylation site and multiple O-glycosylation sites that play a role in its glycosylation. The protein is attached to the cell membrane using a glycosylphosphatidylinositol (GPI) anchor at its end (C-terminus) (30). The CD24 crystal structure was defined using X-ray crystallography, revealing a tight, spherical shape with a β-barrel configuration made of 4 antiparallel β-strands. A disulfide bond between Cys53 and Cys73 provides stability to the β-barrel. The beginning section of the protein showcases a brief α-helix and an adaptable loop area (31).
CD24 and its receptors
CD24 is a cell surface protein that interacts with a range of cell surface receptors, such as P-selectin, Siglec-10, and β1 integrin, and is involved in regulating cell adhesion, migration, cell differentiation, and apoptosis through its association with the Notch signaling pathway (15, 32–34).
While CD24 lacks intrinsic enzymatic activity, it interacts with multiple receptor proteins, such as Siglec-10, Siglec-15, and the NKG2D receptor (35, 36). The Siglec family of receptors, found on immune cells, engage with CD24 in a sialic acid-dependent manner. Siglec-10 functions as a negative regulator of immune responses and inhibits dendritic cells and B cells’ activation when interacting with CD24 (15, 37, 38). Siglec-15, expressed on osteoclasts, interacts with CD24, influencing osteoclast differentiation and bone resorption (39–41). The NKG2D receptor, present on natural killer cells and other immune cells, recognizes stress-induced ligands on tumor and infected cells. CD24 acts as a ligand for NKG2D, suppressing NKG2D-mediated immune responses and facilitating tumor immune evasion (13, 42) (see Figure 2).
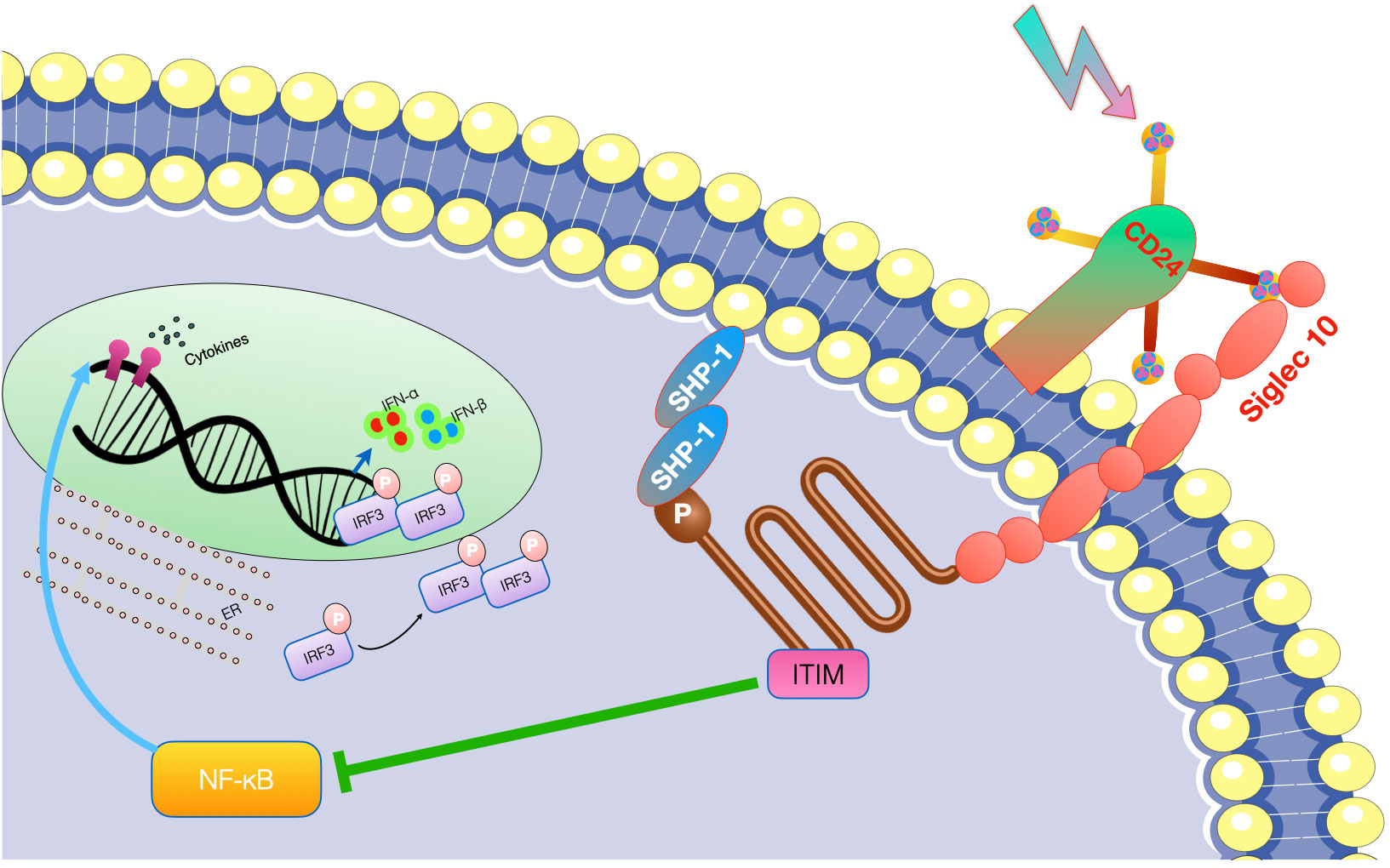
Figure 2 CD24-Siglec-10 pathway as hot targets for tumor immunotherapy. The CD24 antibody has the potential to enhance the immune system’s ability to eliminate tumors. By inhibiting CD24 molecule present on the surface of tumor stem cells, CD24 antibody can block the CD24-Siglec-g/10 signal activation, allowing macrophages to recognize and eliminate tumor cells through the immune clearance process.
In conclusion, CD24 is a cell surface protein involved in critical interactions with various receptors, impacting immune regulation, bone health, and tumor immune evasion. Understanding these CD24 functions and its interactions with receptors is essential for developing CD24-targeted therapies for various diseases.
Role of CD24 in cancer
Studies have shown that CD24 is highly expressed in various tumor cells (21, 43). Recent studies have demonstrated that increased CD24 levels in the blood might be a new prognostic indicator and a biomarker for early cancer detection (18, 44–47). Mechanisms of tumorigenesis promotion by CD24 include cancer stem cell regulation, metastasis of tumor cell, proliferation of cancer cells and evasion of immune detection.
Initially, scientists have introduced the idea of cancer stem cells as the originating precursor cells in the formation of tumors. The association between CD24 and cancer stem cells has been proved in various types of cancer, including breast cancer (48), ovarian cancer (49), pancreatic cancer (50), hepatocellular carcinoma (51), bladder cancer (52), melanoma (53), colon cancer (54), leukemia (55), and multiple myeloma (56). CD24 expression was linked to CSC-like characteristics and the tumorigenic potential of these cells, suggesting that CD24 could serve as a surface marker for CSCs in both solid and hematological tumors; however, the cellular mechanisms of the CD24 - mediated effects are still unclear. Secondly, CD24 plays a crucial role in promoting tumor cell metastasis via multiple mechanisms. By attaching to P-selectin, it diminishes the adhesion of tumor cells, which aids in their movement along the vascular endothelium and platelets, thus increasing their ability to migrate and metastasize (57). CD24 also plays a part in the E-selectin-mediated movement of tumor cells across the surface of vascular endothelium, and it activates integrin subunits, enabling tumor cells to bind to extracellular matrix components and increase their mobility (58). Additionally, CD24 regulates key factors like STAT3 and tissue factor pathway inhibitor-2 (TFPI-2), influencing tumor metastasis by affecting their expression and activity (59, 60). Thirdly, CD24 significantly affects the growth of tumor cells by altering the expression of crucial signaling molecules. Microarray analysis has shown that CD24 mAb downregulates genes associated with carcinogenesis, including MAPK, Ras, and Bcl-2 (61). Additionally, CD24 is capable of initiating ERK and p38MAPK activation, which stimulates the proliferation of tumor cells in both controlled lab environments and living beings. CD24 also regulates the epidermal growth factor receptor (EGFR), a critical player in cell proliferation, by inhibiting its internalization and degradation, thereby affecting cell proliferation (62). Furthermore, the anti-CD24 mAb, G7 mAb, enhances the inhibitory effect of cetuximab on tumor proliferation in vivo, suggesting that CD24 might promotes the growth of tumor cells through the regulation of EGFR expression (63). Fourthly, CD24 plays a critical role in promoting tumor immune evasion through its interaction with Siglec-10. Siglec-10 binds tightly to CD24 in a sialic acid-dependent manner, leading to the inhibition of macrophage signaling cascades and diminished efficiency of phagocytosis, ultimately enhancing tumor immune escape (15). Additionally, elevated expression of Siglec-10 on natural killer (NK) cells correlates with weakened NK cell activity, further making tumors evade the immune system (64). CD24 has been demonstrated to trigger cell death in B cells and precursor B acute lymphoblastic leukemia cells, potentially impacting cellular immunity (65). Siglec-10 inhibits T cell activation, and malignant tumor-derived extracellular vesicles can upregulate Siglec-10 expression in T cells inside tumor microenvironment, decreasing T cell activation. Moreover, correctly glycosylated CD24 can bind to Siglec-10, blocking the activation of T cell receptors by suppressing kinases associated with TCR. These interactions collectively contribute to tumor immune evasion (66, 67) (see Figure 3).
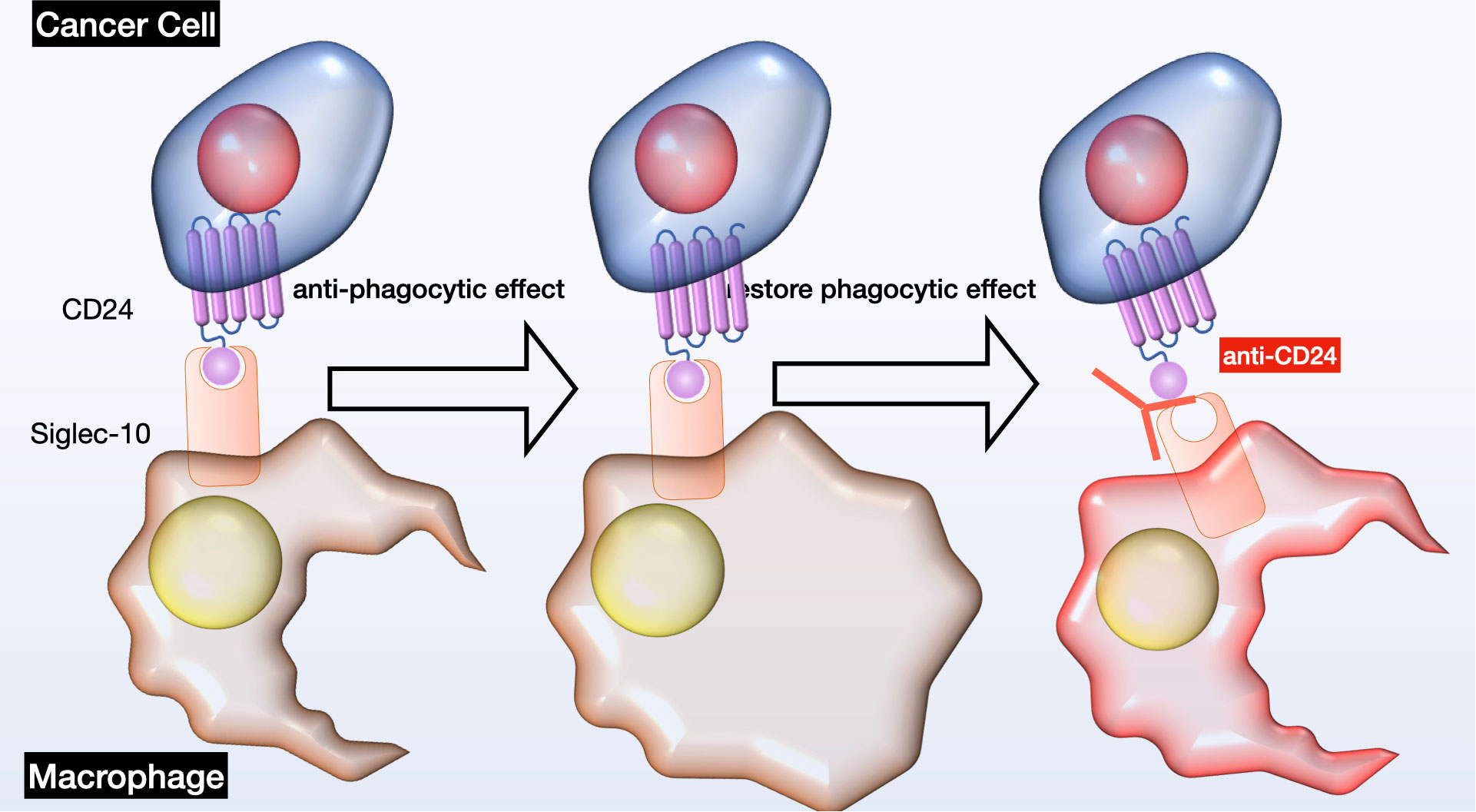
Figure 3 Schematic of CD24-Siglec-10 signaling in cancer immunotherapy. This diagram depicts the inhibitory receptor Siglec-10 identifying and binding to its ligand CD24 on ovarian cancer cells, leading to anti-phagocytic signaling pathways. Intervening with CD24 could potentially reinstate immune cell phagocytosis. OC, ovarian cancer; Siglec, sialic-acid-binding Ig-like lectin.
CD24 plays a pivotal role in promoting tumor growth, metastasis, and immune evasion. Experimental investigations involving the deletion of the CD24 gene and therapeutic interventions have shown significant inhibition of tumor growth in animal models and improved patient survival (15). Consequently, targeting CD24 emerges as a promising therapeutic strategy for cancer treatment. However, it’s noteworthy that the creation of targeted treatments focusing on CD24 is mainly at the preclinical research phase, and comprehensive clinical trial data are currently limited.
To date, there have been two completed clinical trials focused on testing CD24-blocking drugs in cancer patients. The initial trial was a combined Phase 1/2 study involving 58 patients suffering from aggressive B cell lymphoproliferative disorders following bone marrow or organ transplants (68, 69). The participants in the study were administered a dual monoclonal antibody regimen, comprising ALB9 aimed at CD24 and BL13 targeting CD21 (68, 69). Generally, the treatment regimen was well-received, with the primary side effects being temporary neutropenia of grade 3 or higher in 42% of cases, and grade 2 fever in 22% of patients during the initial infusion (68, 69). Instances of grade 3 sepsis, diarrhea, vomiting, and thrombocytopenia were each observed in one patient. The second trial was a phase 1/2 study conducted at a single institution, involving 36 individuals with primary hepatocellular carcinoma who had undergone surgical resection (70). The patients in this study were treated with adjuvant therapy, which included autologous transfusions of dendritic cells and cytokine-induced T cells, both loaded with the CD24 peptide (70). This treatment proved to be safe, with the most frequent side effect being a transient fever of less than grade 3, occurring in 19% of the participants. There were no reported adverse events of grade 3 or higher. After four years, the overall survival rates were 47% and 53% for patients who received the study treatment two and four times, respectively (70).
Though significant progress has been achieved in understanding the functions of CD24 in cancer, research on its role in neural cancers has been relatively limited. For example, while CD24 polymorphisms have been studied in experimental autoimmune encephalomyelitis (EAE) and various cancer types, their examination within the scope of neural cancers has been lacking. Gleaning knowledge from findings in various types of cancer, along with CD24’s complex involvement with cellular communication networks as previously mentioned, ought to provide a more profound comprehension of CD24’s function in the biology of neural tumors. The ability of CD24 on the cell surface to encourage the spread of cancer is associated with its interaction with P-selectin, which is found on stimulated platelets and the cells lining blood vessels (57) (see Figure 4).
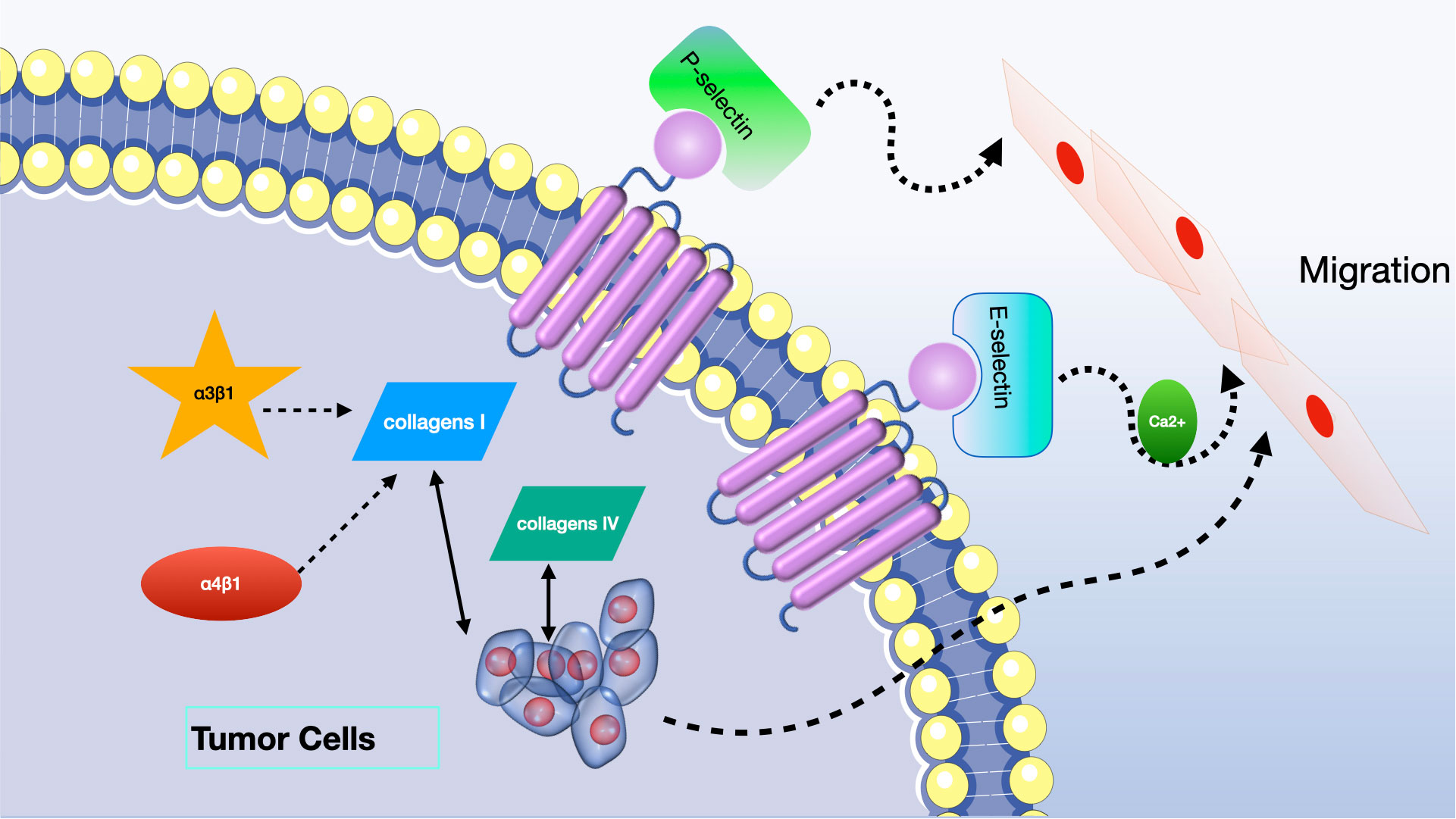
Figure 4 CD24 enhances tumor metastasis by either binding to selectin molecules or activating pre-existing integrin subunits.
CD24 is recognized as a potential indicator for CSCs or cells initiating brain tumors because it is found on the cell surface and is linked to the advancement of metastasis. In solid tumor CSCs of breast cancer, a CD44+/CD24− phenotype has been identified. These CD44+/CD24−/low breast CSCs have demonstrated increased tumorigenic capabilities (48, 71). Additionally, breast cancer cases exhibiting the CD44+/CD24−/low phenotype display poor prognosis and limited response to chemotherapy (72). However, identifying cancer stem cells in this manner has been met with challenges, including conflicting outcomes and considerable heterogeneity within and among various cancer subtypes (73).
CD24 and non-neoplastic disorders
Although CD24 is best known for its involvement in cancer, it also plays a role in various nonneoplastic diseases. It has been implicated in inflammatory and autoimmune diseases, infectious diseases, and neurological disorders. Here, we discuss some of the key roles of CD24 in these nonneoplastic diseases.
Autoimmune diseases
A decade ago, the initial connection between CD24 and autoimmune diseases surfaced when it was discovered that mice lacking CD24 displayed significant resistance to experimental autoimmune encephalomyelitis (22). CD24 is involved in the regulation of the immune response and has been implicated in the pathogenesis of autoimmune diseases. Clinical data provide substantial support for the association between CD24 and autoimmune diseases. CD24 polymorphisms are linked to the progression of autoimmune disorders, including systemic lupus erythematosus (SLE), multiple sclerosis and rheumatoid arthritis (22, 24, 74–76). Within the CD24 gene, there exists a single nucleotide polymorphism (SNP) denoted as P170, which results in a nonconservative alteration in the C-terminus of the mature CD24 protein, either as Alanine (A, P170C) or Valine (A, P170T).
Zhou et al. initially reported that the CD24V/V genotype was associated with an increased risk and progression of multiple sclerosis. They noted that the expression of CD24 on peripheral blood T cells was higher in CD24V/V patients compared to those with the CD24A/A genotype. This association was subsequently validated in a Spanish cohort, although contradictory data were reported by another group from two cohorts (77, 78). In the case of SLE, Sanchez et al. conducted a study involving three Caucasian cohorts from Spain, Germany, and Sweden. They discovered that the prevalence of the CD24V/V genotype was elevated in SLE patients in the Spanish cohort, though this trend was not observed in the German or Swedish cohorts 24). For rheumatoid arthritis, the CD24V/V genotype was found to be more common among patients when a large screening of over a thousand rheumatoid arthritis patients and eight hundred healthy individuals was conducted (76). A similar association was observed in giant cell arthritis (79).
Additionally, there are three other polymorphisms located in the CD24 mRNA long UTR, namely P1056, P1527, and P1626. Among these, the dinucleotide deletion of P1527 has the capacity to destabilize CD24 mRNA and, significantly, this deletion offers protection against both multiple sclerosis and SLE (80).
Multiple sclerosis stands as the most prevalent autoimmune disorder within the central nervous system, characterized by persistent inflammation and extensive damage involving the loss of myelin and axons. The initial onset of MS involves the infiltration of the CNS by self-targeting immune cells, specifically T cells. The incidence of MS has been associated with both environmental and genetic factors (81, 82). CD24, which is under developmental regulation in T cells (7), serves as a co-stimulatory molecule that enhances T cell activation (83). In the experimental model for MS, experimental autoimmune encephalomyelitis (EAE), CD24 is essential for autoreactive T cells as well as resident CNS lymphocytes (22, 75).
The precise mechanism through which CD24 influences autoimmune diseases remains to be fully understood. In addition to its role as a costimulatory factor, CD24 serves as a genetic checkpoint in the context of T-cell homeostatic proliferation, especially in lymphogenic hosts. Lymphopenia, a common occurrence in autoimmune diseases, triggers T-cell homeostatic proliferation. It has been established that CD24 expressed on T cells plays a crucial role in this process (84, 85). Additionally, considering that CD24 influences the efficiency of clonal deletion, it is plausible that mice carrying a specific mutation in CD24 could exhibit a decreased presence of high-affinity autoreactive T cells (86).
Inflammation diseases
Inflammation is a natural immune response to infections and tissue damage. It is triggered by various agents, which can be categorized into two main groups. The primary and most influential category includes molecular patterns related to pathogens, known as PAMPs, while the secondary category, which is of lesser importance, contains molecular patterns associated with damage, known as DAMPs (87, 88).
In the context of inflammatory diseases, CD24 has a multifaceted role, acting as both a facilitator and regulator of inflammation. Its function is highly dependent on the context of the disease and the type of immune cells involved. In initial research, it was observed that the interaction between CD24 and Siglec G/10 plays a role in controlling the inflammatory response to DAMPs but not PAMPs. Subsequently, more and more evidence confirmed the relationship between CD24 and several DAMPs, including heat-shock proteins (HSP), high mobility group box protein 1 (HMGB-1) and nucleolins (89, 90). CD24 interacts with SiglecG in mice and Siglec10 in humans to specifically suppress the host’s reaction to tissue injury. Notably, this mechanism does not interfere with the host’s response to PAMPs (91). As a result, the CD24-SiglecG pathway is suggested to have the ability to differentiate between DAMPs and PAMPs (91) (see Figure 5).
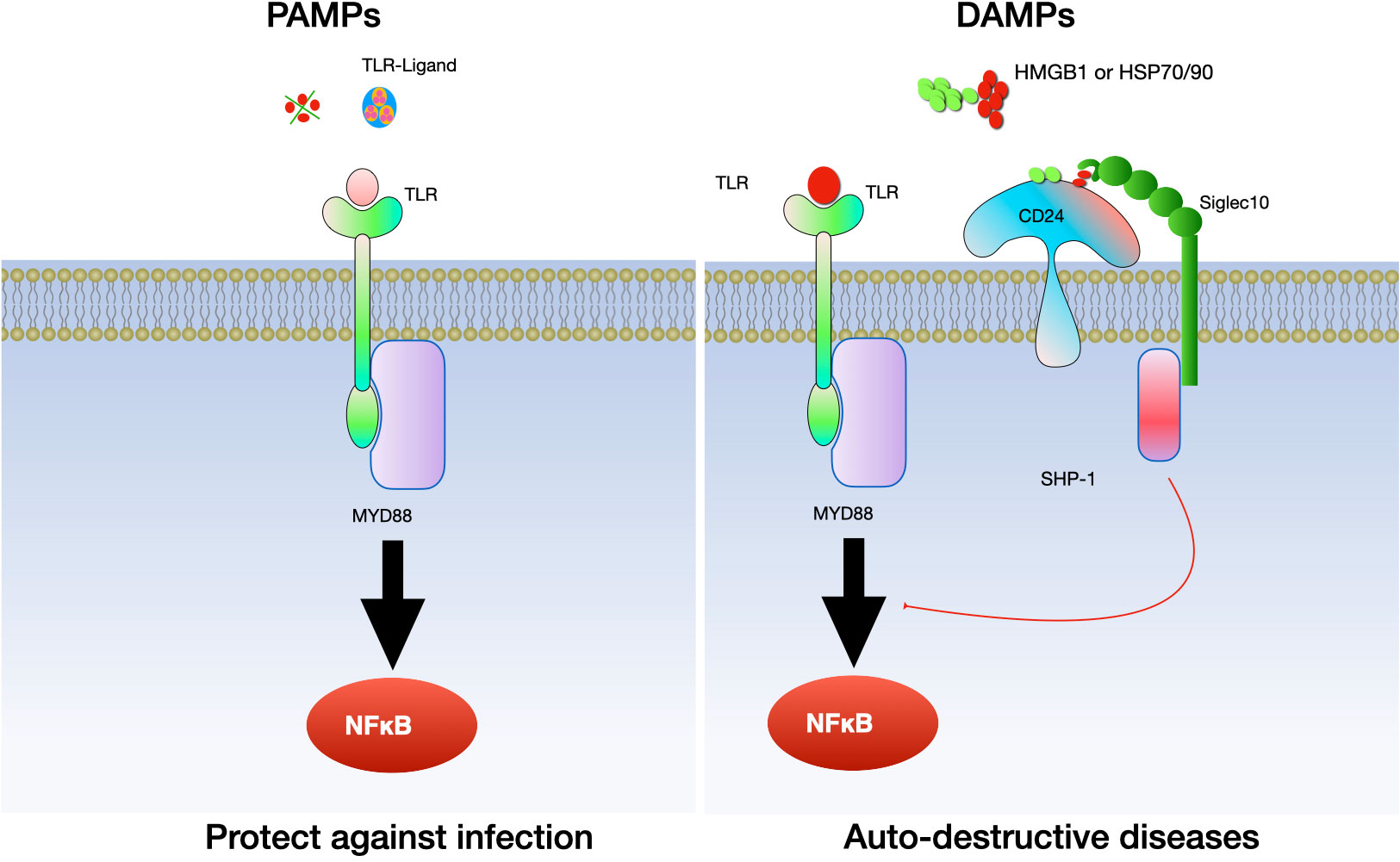
Figure 5 The interaction between CD24 and Siglec 10/G specifically inhibits the inflammatory reaction to tissue damage. CD24 binds with Siglec-10 to suppress inflammatory responses triggered by danger-associated molecular patterns (DAMPs), while it does not affect those elicited by pathogen-associated molecular patterns (PAMPs). Toll-like receptors (TLRs) are involved in this process.
Nevertheless, the response of the host to infectious pathogens can be affected by this interaction, since most infections lead to cell death and could provoke inflammatory reactions via DAMPs. Additionally, numerous pathogens have been discovered to interrupt the CD24-Siglec G/10 connection, either by diminishing the expression of Siglec G/10 or by removing sialic acids from CD24 (25, 92). CD24 has been found to be upregulated in response to viral and bacterial infections, and CD24-deficient mice have been shown to be more susceptible to infection (93–97). Preclinical studies have demonstrated that CD24Fc was effective in protecting non-human primates from acquired immunodeficiency syndrome (AIDS) caused by the simian immunodeficiency virus (98, 99). Human CD24-Fc has been effectively created and experimented with, particularly in rhesus monkeys afflicted with chronic immune issues and inflammation resulting from HIV-1/SIV infection. The treatment was well-received in these tests, suggesting it might help slow the progression to AIDS in SIV-infected primates.
CD24-Fc shows potential as an innovative approach to manage the immune response in diseases marked by persistent immune activation and systemic inflammation. Its effectiveness is currently being further explored in various clinical trials for immune-related conditions such as graft-vs-host disease and other similar disorders. It has been implicated in the regulation of immune responses to infections, including the production of cytokines and chemokines and the recruitment of immune cells to the site of infection (25, 100–103).
CD24 could be proposed to discriminate between DAMPs and PAMPs and deeper understanding CD24-Siglec10 interaction pathways may lead to the development of targeted therapies for managing inflammatory conditions.
Covid-19
Based on the positive results in non-human primates, as well as the safety and clinical performance of CD24Fc in healthy volunteers and patients undergoing bone marrow transplantation, OncoImmune, Inc. initiated a phase 3 clinical trial at nine medical centers in the United States. This study is designed to evaluate the safety and clinical effectiveness of CD24Fc in hospitalized COVID-19 patients requiring oxygen support. The main goal is to measure the duration until clinical improvement, which is marked by the patient’s shift from needing oxygen support to breathing without it over a 28-day observation period 154,.
The data from this clinical trial indicates that CD24Fc is well-received and markedly hastens the rate of clinical recovery by over 60% in hospitalized patients with COVID-19 who require oxygen support. Biomarker studies have revealed that CD24Fc consistently suppresses the inflammatory response in COVID-19 patients. Overall, these results indicate that focusing on inflammation due to tissue damage might provide a treatment possibility for COVID-19 patients in the hospital (100).
In line with the clinical outcomes of CD24Fc, it was observed that HMGB1 (High Mobility Group Box 1) is elevated in the plasma of COVID-19 patients (104, 105). Furthermore, RNA sequencing analysis of lung tissue from both healthy individuals and severe COVID-19 patients showed a selective reduction in SIGLEC10 mRNA expression without affecting the expression of other SIGLECS (92). More recently, a non-randomized study by Shapira et al. suggested that Exo-CD24, which includes CD24-containing exosomes, appeared to reduce inflammatory markers and cytokines/chemokines while expediting the recovery of hospitalized COVID-19 patients (106).
Neurological disorders
CD24 has been found to promote axonal growth and myelination, and CD24-deficient mice have been shown to have impaired neural development (32, 107, 108). Like numerous other surface antigens present on neural cells, CD24 holds significance in cellular communication and function, particularly in processes such as neural migration, the extension of neurites, and neurogenesis (109). Studies indicate dynamic expression of CD24 during the neural development of rodents, and at least one study has suggested transient expression of CD24 during human development (110–112). These findings also emphasize that CD24 could undergo transcriptional activation in postmitotic neurons during the migration phase but is typically lost once a more mature cytoarchitectural context is established. Calaora and colleagues showed that the expression of mCD24 is maintained in certain areas of adult mice that are involved in the secondary formation of neurons (110). Notably, CD24 expression endures into adulthood within the rostral migratory stream, the pathway used by newly formed neurons toward the olfactory bulb, as well as in the dentate gyrus of the hippocampus. These results support the notion that CD24 is present during stages of neuronal migration and the formation of neuronal connections. The observations are consistent with the idea that CD24 functions as a glycoprotein that plays a role in directing neuronal migration and the formation of synapses. Furthermore, CD24 expression was observed in non-neuronal ependymal cells that possess cilia and line the ventricles (113). The precise degree to which CD24’s glycans may distinctively guide the movement of neurons and the creation of connections during this stage of development is still a matter to be determined through research.
CD24 has also been implicated in the pathogenesis of neurological disorders, including neuronal injury, etc. CD24 can enhance neuronal regeneration in experimental subarachnoid hemorrhages (114). Astrocytes have the potential to alleviate neuronal damage by utilizing CD24 to inhibit NF-κB binding activity, thereby reducing the secretion of inflammatory factors subsequent to the HMGB1 challenge (see Figure 5). CD24 appears to enable T cells to evade clonal deletion, a process that deactivates self-reactive cells before maturation, but it does not influence the entry of self-reactive T cells into the CNS (86, 115). The expression of CD24 on resident CNS lymphocytes exacerbates the severity of EAE by boosting the activation of autoreactive T cells (75), crucial for their local multiplication (115). This interaction does not seem to involve a like-to-like trans interaction between CD24 molecules (116). Overall, the cumulative evidence suggests that CD24 expression encourages the development of EAE, and potentially MS, following initiation by various other contributing factors (117).
Metabolic disorder
Metabolic disorders such as obesity, dyslipidemia, diabetes, nonalcoholic fatty liver disease, and nonalcoholic steatohepatitis have significantly emerged as a major global health concern (118). Metaflammation, characterized as a persistent low-grade inflammatory state in metabolic tissues, stands as a significant hallmark of metabolic disease (119). This chronic state of tissue inflammation involves the infiltration and activation of immune cells along with elevated levels of inflammatory cytokines, resulting in impaired insulin signaling and disruption of systemic metabolic balance (120). Several inflammatory signaling pathways like JNK and IKK, alongside inflammatory cytokines such as TNF-α and IL-1β, have been implicated in the development of metabolic diseases (121–124) Despite compelling evidence linking chronic inflammation to obesity, the mechanisms underlying the initiation and control of metaflammation during obesity remain inadequately understood.
Yang L et al. identified that the disruption of the CD24-Siglec-E interaction exacerbates metabolic disorders associated with obesity, while therapy involving CD24Fc shows improvement (26). The recognition of CD24 by Siglec-E through sialoside-based interactions negatively regulates metaflammation and offers protection against metabolic syndrome. Clinical studies on CD24Fc confirm the significance of this pathway in human lipid processing and inflammatory responses. These discoveries highlight the pivotal inhibitory function of the CD24-Siglec-E axis in metabolic dysfunctions and metaflammation, offering a potential immunotherapeutic approach for conditions like obesity, dyslipidemia, insulin resistance, and nonalcoholic steatohepatitis. They also evaluated the role of CD24-Siglec pathway in the development of metabolic syndrome and demonstrated that CD24 deficiency aggravates metabolic disorder in mice. To pinpoint the CD24 receptor involved in metabolic regulation, researchers employed a genetic strategy to ascertain if mutations in any Siglec gene could replicate the metabolic characteristics observed when CD24 is removed. They finally found that Siglec-E signaling is required for CD24-mediated protection against metabolic disorder and CD24-Siglec-E axis represses metaflammation to ameliorate metabolic disorder. Given that CD24Fc is undergoing clinical development for several human diseases, the recent study offers a promising therapeutic strategy for treating metabolic diseases by strengthening sialoside-based pattern recognition (see Figure 6).
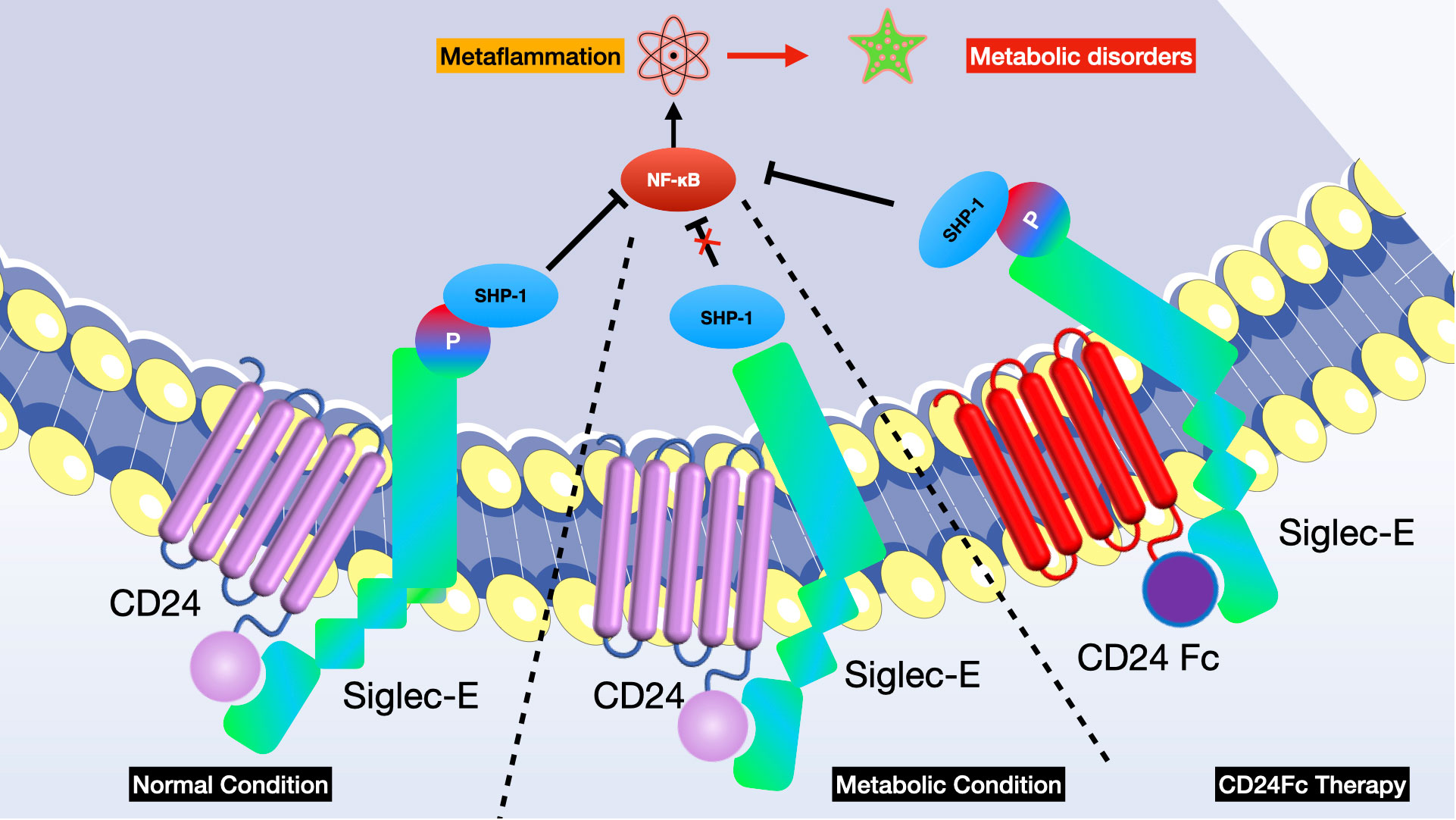
Figure 6 Disruption of the CD24-Siglec-E axis worsens, whereas CD24Fc treatment improves, metabolic disorders associated with obesity. Sialylated CD24 triggers the recruitment of SHP-1 to Siglec-E, thereby reducing metaflammation. In the initial phase I clinical trial conducted on humans, a sole administration of CD24Fc proved safe and well-received among healthy individuals.
Conclusion
CD24 has emerged as a promising therapeutic target in cancer due to its significant role in the disease. Researchers have extensively investigated the use of antibodies that target CD24 for cancer treatment. While preclinical studies exploring CD24 antibody-based therapies have been conducted and reviewed previously (Table 1) (125), it’s worth noting that as of now, there have been no clinical trials targeting CD24 in cancer treatment. While the role of CD24 in cancer has garnered significant attention, its importance extends to various other physiological and pathological processes, including autoimmune diseases, infectious disorders, Covid-19, neurological disorders, metabolic disorders and more. Further research into CD24 functions in these areas may reveal new insights and potential therapeutic applications beyond cancer.
To enhance patient outcomes, it is imperative to tackle the issue of drug resistance mediated by CD24 and prolong patient survival. Nevertheless, the exact mechanisms through which CD24 contributes to drug resistance in tumor cells remain incompletely elucidated, involving a complex interplay of diverse pathways. Clinically, it requires additional assessment to determine how to integrate phagocytosis checkpoint inhibitors and/or activators into the existing framework of immunotherapy. In addition, investigating the potential for combining CD24-targeted immunotherapy with other treatments, such as checkpoint inhibitors or chemotherapy, is a critical area of research. Determining the synergistic effects and potential toxicities of combination therapies is essential. Furthermore, designing well-controlled clinical trials with appropriate endpoints and patient cohorts is essential for robustly evaluating the efficacy of CD24-targeted immunotherapy. Also, Assessing the safety profile of CD24-targeted immunotherapy is crucial. Understanding potential side effects and their management is essential to ensure that the treatment does not cause significant harm to patients. Moreover, identifying predictive biomarkers that can help select patients who are most likely to respond to CD24-targeted immunotherapy is an important consideration. This will help in patient stratification and personalized treatment approaches.
In summary, while CD24-targeted immunotherapy holds promise in the treatment of cancer and non-neoplastic diseases, numerous questions related to its clinical application, efficacy, safety, and combination strategies still need to be addressed through rigorous research and clinical trials.
Author contributions
HW: Software, Writing – original draft. PS: Writing – review & editing, Supervision, Investigation. XS: Data curation, Writing – original draft. YL: Resources, Writing – original draft. HX: Supervision, Writing – review & editing. HZ: Conceptualization, Funding acquisition, Investigation, Supervision, Writing – original draft, Writing – review & editing.
Funding
The author(s) declare financial support was received for the research, authorship, and/or publication of this article. This work was funded by the Shandong Province Natural Science Foundation grants ZR2022QH372.
Conflict of interest
The authors declare that the research was conducted in the absence of any commercial or financial relationships that could be construed as a potential conflict of interest.
Publisher’s note
All claims expressed in this article are solely those of the authors and do not necessarily represent those of their affiliated organizations, or those of the publisher, the editors and the reviewers. Any product that may be evaluated in this article, or claim that may be made by its manufacturer, is not guaranteed or endorsed by the publisher.
References
1. Springer T, Galfrè G, Secher DS, Milstein C. Monoclonal xenogeneic antibodies to murine cell surface antigens: identification of novel leukocyte differentiation antigens. Eur J Immunol (1978) 8:539–51. doi: 10.1002/eji.1830080802
2. Altevogt P, Sammar M, Hüser L, Kristiansen G. Novel insights into the function of CD24: A driving force in cancer. Int J Cancer (2021) 148:546–59. doi: 10.1002/ijc.33249
3. Fang X, Zheng P, Tang J, Liu Y. CD24: from A to Z. Cell Mol Immunol (2010) 7:100–3. doi: 10.1038/cmi.2009.119
4. Pirruccello SJ, LeBien TW. The human B cell-associated antigen CD24 is a single chain sialoglycoprotein. J Immunol (1986) 136:3779–84. doi: 10.4049/jimmunol.136.10.3779
5. Qiao XJ, Gu Y, Du H, Xing ZW, Ma BH, Sun WT, et al. Co-expression of CD24 and Hsp70 as a prognostic biomarker for lung cancer. Neoplasma (2021) 68:1023–32. doi: 10.4149/neo_2021_210118N81
6. Pirruccello SJ, Lang MS. Differential expression of CD24-related epitopes in mycosis fungoides/Sezary syndrome: A potential marker for circulating Sezary cells. Blood (1990) 76:2343–447. doi: 10.1182/blood.v76.11.2343.2343
7. Crispe IN, Bevan MJ. Expression and functional significance of the J11d marker on mouse thymocytes. J Immunol (1987) 138:2013–8. doi: 10.4049/jimmunol.138.7.2013
8. Elghetany MT, Patel J. Assessment of CD24 expression on bone marrow neutrophilic granulocytes: CD24 is a marker for the myelocytic stage of development. Am J Hematol (2002) 71:348–9. doi: 10.1002/ajh.10176
9. Bates ME, Liu LY, Esnault S, Stout BA, Fonkem E, Kung V, et al. Expression of interleukin-5- and granulocyte macrophage-colony-stimulating factor-responsive genes in blood and airway eosinophils. Am J Respir Cell Mol Biol (2004) 30:736–43. doi: 10.1165/rcmb.2003-0234OC
10. Williams LA, Mclellan AD, Summers KL, Sorg RV, Fearnley DH, Hart DNJ. Identification of a novel dendritic cell surface antigen defined by carbohydrate specific CD24 antibody cross-reactivity. Immunology (1996) 89:120–5. doi: 10.1046/j.1365-2567.1996.d01-720.x
11. Hubbe M, Altevogt P. Heat-stable antigen/CD24 on mouse T lymphocytes: evidence for a costimulatory function. Eur J Immunol (1994) 24:731–7. doi: 10.1002/eji.1830240336
12. Wei M, Wang PG. Desialylation in physiological and pathological processes: New target for diagnostic and therapeutic development. Prog Mol Biol Trans Sci (2019), 25–57. doi: 10.1016/bs.pmbts.2018.12.001
13. Panagiotou E, Syrigos NK, Charpidou A, Kotteas E, Vathiotis IA. CD24: A novel target for cancer immunotherapy. J Pers Med (2022) 12:1–15. doi: 10.3390/jpm12081235
14. Altevogt P, Sammar M, Hüser L, Kristiansen G. Novel insights into the function of CD24 : A driving force in cancer. Int J Cancer (2021) 148:546–59. doi: 10.1002/ijc.33249
15. Barkal AA, Brewer RE, Markovic M, Kowarsky M, Barkal SA, Zaro BW, et al. CD24 signalling through macrophage Siglec-10 is a target for cancer immunotherapy. Nature (2019) 572:392–6. doi: 10.1038/s41586-019-1456-0
16. Sagiv E, Starr A, Rozovski U, Khosravi R, Altevogt P, Wang T, et al. Targeting CD24 for treatment of colorectal and pancreatic cancer by monoclonal antibodies or small interfering RNA. Cancer Res (2008) 68:2803–12. doi: 10.1158/0008-5472.CAN-07-6463
17. Tarhriz V, Bandehpour M, Dastmalchi S, Ouladsahebmadarek E, Zarredar H, Eyvazi S. Overview of CD24 as a new molecular marker in ovarian cancer. J Cell Physiol (2019) 234:2134–42. doi: 10.1002/jcp.27581
18. Kristiansen G, Winzer K-J, Mayordomo E, Bellach J, Schlüns K, Denkert C, et al. CD24 expression is a new prognostic marker in breast cancer. Clin Cancer Res (2003) 9:4906–13.
19. Kristiansen G, Pilarsky C, Pervan J, Stürzebecher B, Stephan C, Jung K, et al. CD24 expression is a significant predictor of PSA relapse and poor prognosis in low grade or organ confined prostate cancer. Prostate (2004) 58:183–92. doi: 10.1002/pros.10324
20. Jaggupilli A, Elkord E. Significance of CD44 and CD24 as cancer stem cell markers: An enduring ambiguity. Clin Dev Immunol (2012), 708036. doi: 10.1155/2012/708036
21. Lim SC. CD24 and human carcinoma: Tumor biological aspects. Biomed Pharmacother (2005) 59:S351–4. doi: 10.1016/S0753-3322(05)80076-9
22. Bai XF, Liu JQ, Liu X, Guo Y, Cox K, Wen J, et al. The heat-stable antigen determines pathogenicity of self-reactive T cells in experimental autoimmune encephalomyelitis. J Clin Invest (2000) 105:1227–32. doi: 10.1172/JCI9012
23. Park JH, Kim JH, Jo KE, Na SW, Eisenhut M, Kronbichler A, et al. Field synopsis and re-analysis of systematic meta-analyses of genetic association studies in multiple sclerosis: a Bayesian approach. Mol Neurobiol (2018) 55:5672–88. doi: 10.1007/s12035-017-0773-2
24. Sánchez E, Abelson AK, Sabio JM, Gonzaléz-Gay MA, Ortego-Centeno N, Jiménez-Alonso J, et al. Association of a CD24 gene polymorphism with susceptibility to systemic lupus erythematosus. Arthritis Rheum (2007) 56:3080–6. doi: 10.1002/art.22871
25. Chen GY, Chen X, King S, Cavassani KA, Cheng J, Zheng X, et al. Amelioration of sepsis by inhibiting sialidase-mediated disruption of the CD24-SiglecG interaction. Nat Biotechnol (2011) 29:428–35. doi: 10.1038/nbt.1846
26. Wang X, Liu M, Zhang J, Brown NK, Zhang P, Zhang Y, et al. CD24-Siglec axis is an innate immune checkpoint against metaflammation and metabolic disorder. Cell Metab (2022) 34:1088–103.e6. doi: 10.1016/j.cmet.2022.07.005
27. Liu Y, Zheng P. CD24-Siglec interactions in inflammatory diseases. Front Immunol (2023) 14:1174789. doi: 10.3389/fimmu.2023.1174789
28. Kay R, Rosten PM, Humphries RK. CD24, a signal transducer modulating B cell activation responses, is a very short peptide with a glycosyl phosphatidylinositol membrane anchor. J Immunol (1991) 147:1412–6. doi: 10.4049/jimmunol.147.4.1412
29. Wenger RH, Ayane M, Bose R, Köhler G, Nielsen PJ. The genes for a mouse hematopoietic differentiation marker called the heat-stable antigen. Eur J Immunol (1991) 21:1039–46. doi: 10.1002/eji.1830210427
30. Ayre DC, Pallegar NK, Fairbridge NA, Canuti M, Lang AS, Christian SL. Analysis of the structure, evolution, and expression of CD24, an important regulator of cell fate. Gene (2016) 590:324–37. doi: 10.1016/j.gene.2016.05.038
31. Karnan S, Ota A, Murakami H, Rahman ML, Hasan MN, Wahiduzzaman M, et al. Identification of CD24 as a potential diagnostic and therapeutic target for Malignant pleural mesothelioma. Cell Death Discov (2020) 6:127. doi: 10.1038/s41420-020-00364-1
32. Sammar M, Aigner S, Altevogt P. Heat-stable antigen (mouse CD24) in the brain: Dual but distinct interaction with P-selectin and L1. Biochim Biophys Acta - Protein Structure Mol Enzymol (1997) 1337:287–94. doi: 10.1016/S0167-4838(96)00177-X
33. Pruszak J, Menon V, Pruszak J. The CD24 surface antigen in neural development and disease. Neurobiol Dis (2017) 99:133–44. doi: 10.1016/j.nbd.2016.12.011
34. Pei Z, Zhu G, Huo X, Gao L, Liao S, He J, et al. CD24 promotes the proliferation and inhibits the apoptosis of cervical cancer cells in vitro. Oncol Rep (2016) 35:1593–601. doi: 10.3892/or.2015.4521
35. Jiang KY, Qi LL, Kang FB, Wang L. The intriguing roles of Siglec family members in the tumor microenvironment. biomark Res (2022) 10:22. doi: 10.1186/s40364-022-00369-1
36. Guo Z, Hu J. Research progress and prospect of Siglec in innate immune cells in tumor. China Oncol (2022) 32:1235–41. doi: 10.19401/j.cnki.1007-3639.2022.12.012
37. Yin SS, Gao FH. Molecular mechanism of tumor cell immune escape mediated by CD24/siglec-10. Front Immunol (2020) 11:1324. doi: 10.3389/fimmu.2020.01324
38. Aroldi A, Mauri M, Parma M, Terruzzi E, Fedele M, Perfetti P, et al. CD24/siglec-10 “Don’t eat me” Signal blockade is a potential immunotherapeutic target in mantle-cell lymphoma. Blood (2021) 138:2276. doi: 10.1182/blood-2021-154086
39. Sun J, Lu Q, Sanmanmed MF, Wang J. Siglec-15 as an emerging target for next-generation cancer immunotherapy. Clin Cancer Res (2021) 27:680–88. doi: 10.1158/1078-0432.CCR-19-2925
40. Angata T. Siglec-15: A potential regulator of osteoporosis, cancer, and infectious diseases. J BioMed Sci (2020) 27:10. doi: 10.1186/s12929-019-0610-1
41. Fan M, Zhang G, Xie M, Liu X, Zhang Q, Wang L. Siglec-15 as a new perspective therapy target in human giant cell tumor of bone. Curr Oncol (2022) 29:7655–71. doi: 10.3390/curroncol29100605
42. Han Y, Sun F, Zhang X, Wang T, Jiang J, Cai J, et al. CD24 targeting bi-specific antibody that simultaneously stimulates NKG2D enhances the efficacy of cancer immunotherapy. J Cancer Res Clin Oncol (2019) 145:1179–90. doi: 10.1007/s00432-019-02865-8
43. Aroldi A, Mauri M, Ramazzotti D, Villa M, Malighetti F, Crippa V, et al. Effects of blocking CD24 and CD47 ‘don’t eat me’signals in combination with rituximab in mantle-cell lymphoma and chronic lymphocytic leukaemia. J Cell Mol Med (2023) 27:3053–64. doi: 10.1111/jcmm.17868
44. Kristiansen G, Schlüns K, Yongwei Y, Denkert C, Dietel M, Petersen I. CD24 is an independent prognostic marker of survival in nonsmall cell lung cancer patients. Br J Cancer (2003) 88:231–6. doi: 10.1038/sj.bjc.6600702
45. Kristiansen G, Denkert C, Schlüns K, Dahl E, Pilarsky C, Hauptmann S. CD24 is expressed in ovarian cancer and is a new independent prognostic marker of patient survival. Am J Pathol (2002). doi: 10.1016/S0002-9440(10)64398-2
46. Weichert W, Denkert C, Burkhardt M, Gansukh T, Bellach J, Altevogt P, et al. Cytoplasmic CD24 expression in colorectal cancer independently correlates with shortened patient survival. Clin Cancer Res (2005) 11:6574–81. doi: 10.1158/1078-0432.CCR-05-0606
47. Agrawal S, Kuvshinoff BW, Khoury T, Yu J, Javle MM, LeVea C, et al. CD24 expression is an independent prognostic marker in Cholangiocarcinoma. J Gastrointestinal Surg (2007) 11:445–51. doi: 10.1007/s11605-007-0091-5
48. Al-Hajj M, Wicha MS, Benito-Hernandez A, Morrison SJ, Clarke MF. Prospective identification of tumorigenic breast cancer cells. Proc Natl Acad Sci USA (2003) 100:3983–8. doi: 10.1073/pnas.0530291100
49. Klemba A, Purzycka-Olewiecka JK, Wcisło G, Czarnecka AM, Lewicki S, Lesyng B, et al. Surface markers of cancer stem-like cells of ovarian cancer and their clinical relevance. Wspolczesna Onkologia (2017) 22:48–55. doi: 10.5114/wo.2018.73885
50. Abel EV, Simeone DM. Biology and clinical applications of pancreatic cancer stem cells. Gastroenterology (2013) 144:1241–8. doi: 10.1053/j.gastro.2013.01.072
51. Lee TKW, Castilho A, Cheung VCH, Tang KH, Ma S, Ng IOL. CD24(+) liver tumor-initiating cells drive self-renewal and tumor initiation through STAT3-mediated NANOG regulation. Cell Stem Cell (2011) 9:50–63. doi: 10.1016/j.stem.2011.06.005
52. Jinesh GG, Manyam GC, Mmeje CO, Baggerly KA, Kamat AM. Surface PD-L1, E-cadherin, CD24, and VEGFR2 as markers of epithelial cancer stem cells associated with rapid tumorigenesis. Sci Rep (2017) 7:9602. doi: 10.1038/s41598-017-08796-z
53. Tang MR, Guo JY, Wang D, Xu N. Identification of CD24 as a marker for tumorigenesis of melanoma. Onco Targets Ther (2018) 11:3401–3406. doi: 10.2147/OTT.S157043
54. Vermeulen L, Todaro M, De Sousa Mello F, Sprick MR, Kemper K, Perez Alea M, et al. Single-cell cloning of colon cancer stem cells reveals a multi-lineage differentiation capacity. Proc Natl Acad Sci USA (2008) 105:13427–32. doi: 10.1073/pnas.0805706105
55. Perry JM, Tao F, Roy A, Lin T, He XC, Chen S, et al. Overcoming Wnt–β-catenin dependent anticancer therapy resistance in leukaemia stem cells. Nat Cell Biol (2020) 22:689–700. doi: 10.1038/s41556-020-0507-y
56. Gao M, Bai H, Jethava Y, Wu Y, Zhu Y, Yang Y, et al. Identification and characterization of tumor-initiating cells in multiple myeloma. J Natl Cancer Inst (2020) 112:507–15. doi: 10.1093/jnci/djz159
57. Aigner S, Ramos CL, Hafezi-Moghadam A, Lawrence MB, Friederichs J, Altevogt P, et al. CD24 mediates rolling of breast carcinoma cells on P-selectin. FASEB J (1998) 12:1241–51. doi: 10.1096/fasebj.12.12.1241
58. Myung JH, Gajjar KA, Pearson RM, Launiere CA, Eddington DT, Hong S. Direct measurements on CD24-mediated rolling of human breast cancer MCF-7 cells on E-selectin. Anal Chem (2011) 83:1078–83. doi: 10.1021/ac102901e
59. Bretz NP, Salnikov AV, Perne C, Keller S, Wang X, Mierke CT, et al. CD24 controls Src/STAT3 activity in human tumors. Cell Mol Life Sci (2012) 69:3863–79. doi: 10.1007/s00018-012-1055-9
60. Bretz N, Noske A, Keller S, Erbe-Hofmann N, Schlange T, Salnikov AV, et al. CD24 promotes tumor cell invasion by suppressing tissue factor pathway inhibitor-2 (TFPI-2) in a c-Src-dependent fashion. Clin Exp Metastasis (2012) 29:27–38. doi: 10.1007/s10585-011-9426-4
61. Wang W, Wang X, Peng L, Deng Q, Liang Y, Qing H, et al. CD24-dependent MAPK pathway activation is required for colorectal cancer cell proliferation. Cancer Sci (2010) 101:112–9. doi: 10.1111/j.1349-7006.2009.01370.x
62. Deng W, Gu L, Li X, Zheng J, Zhang Y, Duan B, et al. CD24 associates with EGFR and supports EGF/EGFR signaling via RhoA in gastric cancer cells. J Transl Med (2016) 14:32. doi: 10.1186/s12967-016-0787-y
63. Chen Z, Wang T, Tu X, Xie W, He H, Wang M, et al. Antibody-based targeting of CD24 enhances antitumor effect of cetuximab via attenuating phosphorylation of Src/STAT3. Biomed Pharmacother (2017) 90:427–36. doi: 10.1016/j.biopha.2017.03.094
64. Zhang P, Lu X, Tao K, Shi L, Li W, Wang G, et al. Siglec-10 is associated with survival and natural killer cell dysfunction in hepatocellular carcinoma. J Surg Res (2015) 194:107–13. doi: 10.1016/j.jss.2014.09.035
65. Suzuki T, Kiyokawa N, Taguchi T, Sekino T, Katagiri YU, Fujimoto J. CD24 induces apoptosis in human B cells via the glycolipid-enriched membrane domains/rafts-mediated signaling system. J Immunol (2001) 166:5567–77. doi: 10.4049/jimmunol.166.9.5567
66. Bandala-Sanchez E, Zhang Y, Reinwald S, Dromey JA, Lee BH, Qian J, et al. T cell regulation mediated by interaction of soluble CD52 with the inhibitory receptor Siglec-10. Nat Immunol (2013) 14:741–8. doi: 10.1038/ni.2610
67. Li Y, Zhou J, Zhuo Q, Zhang J, Xie J, Han S, et al. Malignant ascite-derived extracellular vesicles inhibit T cell activity by upregulating Siglec-10 expression. Cancer Manag Res (2019) 11:7123–34. doi: 10.2147/CMAR.S210568
68. Fischer A-M, Blanche S, Le Bidois J, Bordigoni P, Garnier JL, Niaudet P, et al. Anti-B-cell monoclonal antibodies in the treatment of severe B-cell lymphoproliferative syndrome following bone marrow and organ transplantation. N Engl J Med (1991) 324:1451–6. doi: 10.1056/NEJM199105233242102
69. Benkerrou M, Jais JP, Leblond V, Durandy A, Sutton L, Bordigoni P, et al. Anti-B-cell monoclonal antibody treatment of severe posttransplant B-lymphoproliferative disorder: prognostic factors and long-term outcome. Blood (1998) 92:3137–47. doi: 10.1182/blood.v92.9.3137
70. Xu K, Meng Z, Mu X, Sun B, Chai Y. One single site clinical study: to evaluate the safety and efficacy of immunotherapy with autologous dendritic cells, cytokine-induced killer cells in primary hepatocellular carcinoma patients. Front Oncol (2020) 10:581270. doi: 10.3389/fonc.2020.581270
71. Zuoren Y, Timothy G, Michael P, et al. Cancer stem cells. (2012) 44:2144–51. doi: 10.1016/j.biocel.2012.08.022
72. Chen Y, Song J, Jiang Y, Yu C, Ma Z. Predictive value of CD44 and CD24 for prognosis and chemotherapy response in invasive breast ductal carcinoma. Int J Clin Exp Pathol (2015) 8:11287–95.
73. Magee JA, Piskounova E, Morrison SJ. Cancer stem cells: impact, heterogeneity, and uncertainty. Cancer Cell (2012) 21:283–96. doi: 10.1016/j.ccr.2012.03.003
74. Zhou Q, Rammohan K, Lin S, Robinson N, Li O, Liu X, et al. CD24 is a genetic modifier for risk and progression of multiple sclerosis. Proc Natl Acad Sci USA (2003) 100:15041–6. doi: 10.1073/pnas.2533866100
75. Liu J-Q, Carl JW, Joshi PS, RayChaudhury A, Pu X-A, Shi F-D, et al. CD24 on the resident cells of the central nervous system enhances experimental autoimmune encephalomyelitis. J Immunol (2007) 178:6227–35. doi: 10.4049/jimmunol.178.10.6227
76. Sánchez E, Fernández-Gutierrez B, González-Gay MÁ, Balsa A, García A, Rodríguez L, et al. Investigating the role of CD24 gene polymorphisms in rheumatoid arthritis. Ann Rheum Dis (2008) 67:1197–8. doi: 10.1136/ard.2007.084475
77. Otaegui D, Sáenz A, Camaño P, Blázquez L, Goicoechea M, Ruíz-Martínez J, et al. CD24 V/V is an allele associated with the risk of developing multiple sclerosis in the Spanish population. Multiple Sclerosis J (2006) 12:511–4. doi: 10.1191/135248506ms1314sr
78. Goris A, Maranian M, Walton A, Yeo TW, Ban M, Gray J, et al. CD24 Ala/Val polymorphism and multiple sclerosis. J Neuroimmunol (2006) 175:200–2. doi: 10.1016/j.jneuroim.2006.03.009
79. Rueda B, Miranda-Filloy JA, Martin J, Gonzalez-Gay MA. Association of CD24 gene polymorphisms with susceptibility to biopsy-proven giant cell arteritis. J Rheumatol (2008) 35:850–4.
80. Wang L, Lin S, Rammohan KW, Liu Z, Liu JQ, Liu RH, et al. A dinucleotide deletion in CD24 confers protection against autoimmune diseases. PloS Genet (2007) 3:e49. doi: 10.1371/journal.pgen.0030049
81. Dobson R, Giovannoni G. Multiple sclerosis – a review. Eur J Neurol (2019) 26:27–40. doi: 10.1111/ene.13819
82. Yang JH, Rempe T, Whitmire N, Dunn-Pirio A, Graves JS. Therapeutic advances in multiple sclerosis. Front Neurol (2022) 13:824926. doi: 10.3389/fneur.2022.824926
83. Liu Y, Jones B, Aruffo A, Sullivan KM, Linsley PS, Janeway CA. Heat-stable antigen is a costimulatory molecule for CD4 T cell growth. J Exp Med (1992) 175:437–45. doi: 10.1084/jem.175.2.437
84. Christian SL. CD24 as a potential therapeutic target in patients with B-cell leukemia and lymphoma: current insights. Onco Targets Ther (2022) 15:1391–402. doi: 10.2147/OTT.S366625
85. Zhang X, Yu C, Liu JQ, Bai XF. Dendritic cell expression of CD24 contributes to optimal priming of T lymphocytes in lymph nodes. Front Immunol (2023) 14:1116749. doi: 10.3389/fimmu.2023.1116749
86. Carl JW, Liu J-Q, Joshi PS, El-Omrani HY, Yin L, Zheng X, et al. Autoreactive T cells escape clonal deletion in the thymus by a CD24-dependent pathway. J Immunol (2008) 181:320–8. doi: 10.4049/jimmunol.181.1.320
87. Janeway CA, Medzhitov R. Innate immune recognition. Annu Rev Immunol (2002) 20:197–216. doi: 10.1146/annurev.immunol.20.083001.084359
88. Harris HE, Raucci A. Alarmin(g) news about danger: Workshop on Innate Danger Signals and HMGB1. EMBO Rep (2006) 7:774–8. doi: 10.1038/sj.embor.7400759
89. Chen GY, Tang J, Zheng P, Liu Y. CD24 and siglec-10 selectively repress tissue damage - Induced immune responses. Sci (1979) (2009) 323:1722–5. doi: 10.1126/science.1168988
90. Parthasarathy U, Martinelli R, Vollmann EH, Best K, Therien AG. The impact of DAMP-mediated inflammation in severe COVID-19 and related disorders. Biochem Pharmacol (2022) 195:114847. doi: 10.1016/j.bcp.2021.114847
91. Liu Y, Chen GY, Zheng P. CD24-Siglec G/10 discriminates danger- from pathogen-associated molecular patterns. Trends Immunol (2009) 30:557–61. doi: 10.1016/j.it.2009.09.006
92. Blanco-Melo D, Nilsson-Payant BE, Liu WC, Uhl S, Hoagland D, Møller R, et al. Imbalanced host response to SARS-CoV-2 drives development of COVID-19. Cell (2020) 181:1036–45.e9. doi: 10.1016/j.cell.2020.04.026
93. Ahmed MAH, Jackson D, Seth R, Robins A, Lobo DN, Tomlinson IPM, et al. CD24 is upregulated in inflammatory bowel disease and stimulates cell motility and colony formation. Inflamm Bowel Dis (2010) 16:795–803. doi: 10.1002/ibd.21134
94. Parlato M, Souza-Fonseca-Guimaraes F, Philippart F, Misset B, Adib-Conquy M, Cavaillon J. CD24-mediated neutrophil death in inflammation: ex vivo study suggesting a potential role in sepsis. Crit Care (2012) 16:P81. doi: 10.1186/cc11768
95. Zheng X, Wang P, Song J, Tang Y, Xie Y, Jin X, et al. Soluble CD24 is an inflammatory biomarker in early and seronegative rheumatoid arthritis. Ann Med (2023) 55:2246370. doi: 10.1080/07853890.2023.2246370
96. Kedarinath K, Fox CR, Crowgey E, Mazar J, Phelan P, Westmoreland TJ, et al. CD24 expression dampens the basal antiviral state in human neuroblastoma cells and enhances permissivity to Zika virus infection. Viruses (2022) 14:1735. doi: 10.3390/v14081735
97. Mazar J, Li Y, Rosado A, Phelan P, Kedarinath K, Parks GD, et al. Zika virus as an oncolytic treatment of human neuroblastoma cells requires CD24. PLoS One (2018) 13:e0200358. doi: 10.1371/journal.pone.0200358
98. Tian RR, Zhang MX, Liu M, Fang X, Li D, Zhang L, et al. CD24Fc protects against viral pneumonia in simian immunodeficiency virus-infected Chinese rhesus monkeys. Cell Mol Immunol (2020) 17:887–8. doi: 10.1038/s41423-020-0452-5
99. Tian RR, Zhang MX, Zhang LT, Zhang P, Ma JP, Liu M, et al. CD24 and Fc fusion protein protects SIVmac239-infected Chinese rhesus macaque against progression to AIDS. Antiviral Res (2018) 157:9–17. doi: 10.1016/j.antiviral.2018.07.004
100. Song NJ, Allen C, Vilgelm AE, Riesenberg BP, Weller KP, Reynolds K, et al. Treatment with soluble CD24 attenuates COVID-19-associated systemic immunopathology. J Hematol Oncol (2022) 15:5. doi: 10.1186/s13045-021-01222-y
101. Li D, Zheng L, Jin L, Zhou Y, Li H, Fu J, et al. CD24 polymorphisms affect risk and progression of chronic hepatitis B virus infection. Hepatology (2009) 50:735–42. doi: 10.1002/hep.23047
102. Sun H, Pan Y, Wu R, Lv J, Chi X, Wang X, et al. CD24 Ala57Val polymorphism is associated with spontaneous viral clearance in the HCV-infected Chinese population. Liver Int (2015) 35:786–94. doi: 10.1111/liv.12506
103. Lior S, Ilana N, Diana K, Ezra B, Meital S, et al. A dinucleotide deletion in the CD24 gene is a potential risk factor for colorectal cancer. Am Surg (2020) 86:480–5. doi: 10.1177/0003134820919737
104. Petrarca L, Manganelli V, Nenna R, Frassanito A, Ben David S, Mancino E, et al. HMGB1 in pediatric COVID-19 infection and MIS-C: A pilot study. Front Pediatr (2022) 10:868269. doi: 10.3389/fped.2022.868269
105. Chen R, Huang Y, Quan J, Liu J, Wang H, Billiar TR, et al. HMGB1 as a potential biomarker and therapeutic target for severe COVID-19. Heliyon (2020) 6:e05672. doi: 10.1016/j.heliyon.2020.e05672
106. Shapira S, Ben Shimon M, Hay-Levi M, Shenberg G, Choshen G, Bannon L, et al. A novel platform for attenuating immune hyperactivity using EXO-CD24 in COVID-19 and beyond. EMBO Mol Med (2022) 14:e15997. doi: 10.15252/emmm.202215997
107. Kleene R, Yang H, Kutsche M, Schachner M. The neural recognition molecule L1 is a sialic acid-binding lectin for CD24, which induces promotion and inhibition of neurite outgrowth. J Biol Chem (2001) 276:21656–63. doi: 10.1074/jbc.M101790200
108. Lieberoth A, Splittstoesser F, Katagihallimath N, Jakovcevski I, Loers G, Ranscht B, et al. Lewis x and α2,3-sialyl glycans and their receptors TAG-1, contactin, and L1 mediate CD24-dependent neurite outgrowth. J Neurosci (2009) 20:6677–90. doi: 10.1523/JNEUROSCI.4361-08.2009
109. Pruszak J, Ludwig W, Blak A, Alavian K, Isacson O. CD15, CD24, and CD29 define a surface biomarker code for neural lineage differentiation of stem cells. Stem Cells (2009) 27:2928–40. doi: 10.1002/stem.211
110. Calaora V, Chazal G, Nielsen PJ, Rougon G, Moreau H. mCD24 expression in the developing mouse brain and in zones of secondary neurogenesis in the adult. Neuroscience (1996) 73:581–94. doi: 10.1016/0306-4522(96)00042-5
111. Shewan D, Calaora V, Nielsen P, Cohen J, Rougon G, Moreau H. mCD24, a glycoprotein transiently expressed by neurons, is an inhibitor of neurite outgrowth. J Neurosci (1996) 16:2624–34. doi: 10.1523/jneurosci.16-08-02624.1996
112. Poncet C, Frances V, Gristina R, Scheiner C, Pellissier JF, Figarella-Branger D. CD24, a glycosylphosphatidylinositol-anchored molecule, is transiently expressed during the development of human central nervous system and is a marker of human neural cell lineage tumors. Acta Neuropathol (1996) 91:400–8. doi: 10.1007/s004010050442
113. Guirao B, Meunier A, Mortaud S, Aguilar A, Corsi JM, Strehl L, et al. Coupling between hydrodynamic forces and planar cell polarity orients mammalian motile cilia. Nat Cell Biol (2010) 12:341–50. doi: 10.1038/ncb2040
114. Chen XX, Tao T, Gao S, Wang H, Zhou XM, Gao YY, et al. Knock-down of CD24 in astrocytes aggravates oxyhemoglobin-induced hippocampal neuron impairment. Neurochem Res (2022) 47:590–600. doi: 10.1007/s11064-021-03468-x
115. Bai X-F, Li O, Zhou Q, Zhang H, Joshi PS, Zheng X, et al. CD24 controls expansion and persistence of autoreactive T cells in the central nervous system during experimental autoimmune encephalomyelitis. J Exp Med (2004) 200:447–58. doi: 10.1084/jem.20040131
116. Zhou Q, Wu Y, Nielsen PJ, Liu Y. Homotypic interaction of the heat-stable antigen is not responsible for its co-stimulatory activity for T cell clonal expansion. Eur J Immunol (1997) 27:2524–8. doi: 10.1002/eji.1830271009
117. Baek J, Kang S, Byeon H, Woo Hwang K, Min H. Contribution of CD24 polymorphisms to autoimmune disease: A meta-analysis. Comput Biol Med (2015) 64:268–75. doi: 10.1016/j.compbiomed.2015.07.012
118. Kivimäki M, Bartolomucci A, Kawachi I. The multiple roles of life stress in metabolic disorders. Nat Rev Endocrinol (2023) 19:10–27. doi: 10.1038/s41574-022-00746-8
119. Xiong P, Zhang F, Liu F, Zhao J, Huang X, Luo D, et al. Metaflammation in glucolipid metabolic disorders: Pathogenesis and treatment. Biomed Pharmacother (2023) 161:114545. doi: 10.1016/j.biopha.2023.114545
120. Lumeng CN, Saltiel AR. Inflammatory links between obesity and metabolic disease. J Clin Invest (2011) 121:2111–7. doi: 10.1172/JCI57132
121. Hirosumi J, Tuncman G, Chang L, Görgün CZ, Uysal KT, Maeda K, et al. A central, role for JNK in obesity and insulin resistance. Nature (2002) 420:333–6. doi: 10.1038/nature01137
122. Hotamisligil GS, Shargill NS, Spiegelman BM. Adipose expression of tumor necrosis factor-a: Direct role in obesity-linked insulin resistance. Sci (1993) 259:87–91. doi: 10.1126/science.7678183
123. Maedler K, Sergeev P, Ris F, Oberholzer J, Joller-Jemelka HI, Spinas GA, et al. Glucose-induced β cell production of IL-1β contributes to glucotoxicity in human pancreatic islets. J Clin Invest (2002) 110:851–60. doi: 10.1172/jci15318
124. Yuan M, Konstantopoulos N, Lee J, Hansen L, Li ZW, Karin M, et al. Reversal of obesity- and diet-induced insulin resistance with salicylates or targeted disruption of Ikkβ. Sci (1979) (2001) 293:1673–7. doi: 10.1126/science.1061620
Keywords: CD24, CD47, innate checkpoint, non-neoplastic, autoimmune diseases, Covid-19, neurological disease, metabolic diseases
Citation: Wang H, Shi P, Shi X, Lv Y, Xie H and Zhao H (2024) Surprising magic of CD24 beyond cancer. Front. Immunol. 14:1334922. doi: 10.3389/fimmu.2023.1334922
Received: 08 November 2023; Accepted: 28 December 2023;
Published: 19 January 2024.
Edited by:
Zhendong Jin, Second Military Medical University, ChinaReviewed by:
Deyu Zhang, Second Military Medical University, ChinaMonika Holubová, Charles University, Czechia
Andrea Aroldi, University of Milano Bicocca, Italy
Copyright © 2024 Wang, Shi, Shi, Lv, Xie and Zhao. This is an open-access article distributed under the terms of the Creative Commons Attribution License (CC BY). The use, distribution or reproduction in other forums is permitted, provided the original author(s) and the copyright owner(s) are credited and that the original publication in this journal is cited, in accordance with accepted academic practice. No use, distribution or reproduction is permitted which does not comply with these terms.
*Correspondence: Hai Zhao, yidao@qdu.edu.cn