The protective effects of flavonoids and carotenoids against diabetic complications—A review of in vivo evidence
- 1Leicester School of Allied Health Sciences, Faculty of Health & Life Sciences, De Montfort University, Leicester, United Kingdom
- 2Leicester School of Pharmacy, Faculty of Health & Life Sciences, De Montfort University, Leicester, United Kingdom
Diabetes mellitus is a chronic metabolic disorder caused either by inadequate insulin secretion, impaired insulin function, or both. Uncontrolled diabetes is characterized by hyperglycemia which over time leads to fatal damage to both macro-and microvascular systems, causing complications such as cardiovascular diseases, retinopathy and nephropathy. Diabetes management is conventionally delivered through modifications of diet and lifestyle and pharmacological treatment, using antidiabetic drugs, and ultimately insulin injections. However, the side effects and financial cost of medications often reduce patient compliance to treatment, negatively affecting their health outcomes. Natural phytochemicals from edible plants such as fruits and vegetables (F&V) and medicinal herbs have drawn a growing interest as potential therapeutic agents for treating diabetes and preventing the onset and progression of diabetic complications. Flavonoids, the most abundant polyphenols in the human diet, have shown antidiabetic effects in numerous in vitro and preclinical studies. The underlying mechanisms have been linked to their antioxidant, anti-inflammatory and immunomodulatory activities. Carotenoids, another major group of dietary phytochemicals, have also shown antidiabetic potential in recent in vitro and in vivo experimental models, possibly through a mechanism of action similar to that of flavonoids. However, scientific evidence on the efficacy of these phytochemicals in treating diabetes or preventing the onset and progression of its complications in clinical settings is scarce, which delays the translation of animal study evidence to human applications and also limits the knowledge on their modes of actions in diabetes management. This review is aimed to highlight the potential roles of flavonoids and carotenoids in preventing or ameliorating diabetes-related complications based on in vivo study evidence, i.e., an array of preclinical animal studies and human intervention trials. The current general consensus of the underlying mechanisms of action exerted by both groups of phytochemicals is that their anti-inflammatory action is key. However, other potential mechanisms of action are considered. In total, 50 in vivo studies were selected for a review after a comprehensive database search via PubMed and ScienceDirect from January 2002 to August 2022. The key words used for analysis are type-2 diabetes (T2DM), diabetic complications, flavonoids, carotenoids, antioxidant, anti-inflammatory, mechanisms of prevention and amelioration, animal studies and human interventions.
1. Introduction
Diabetes is a chronic endocrine disease caused by either insulin deficiency, ineffective use of insulin or both (1). Diabetes is characterized by prolonged high blood glucose levels (hyperglycemia), which, if left uncontrolled, can result in serious damage to different tissues and organs, e.g., the eyes, kidneys and heart. Diabetes is conventionally classified into two types, i.e., type-I (insulin-dependent) diabetes and type-II (non-insulin dependent) diabetes (1). The current global prevalence of diabetes is estimated to be 9.3% (463 million people). It is projected to increase by 25% in 2030 and 51% in 2045, where regions with economies moving from low-to middle-income status will likely be mostly affected (2).
Type-II diabetes mellitus (T2DM) is the prevalent form of diabetes (around 90%) worldwide (3). Its pathogenesis features a lowered sensitivity of the insulin receptors on cells in the liver, skeletal muscles and adipose tissues, i.e., insulin resistance, resulting in a reduced uptake of glucose into its recipient tissues. This often triggers an initial increased insulin secretion (hyperinsulinemia) from beta-cells in pancreas, which however leads to beta-cell dysfunction at advanced stages with reduced insulin secretion (hypoinsulinemia), ultimately resulting in high blood sugar levels (hyperglycemia) (1). Type-2 diabetic patients are often found obese—80% prevalence rate. The treatment target for T2DM is to re-establish normal blood glucose and lipid levels (4).
Ineffective management of diabetes can lead to many complications, commonly cardiovascular diseases, kidney disease, nerve and eye damage, skin problems, increased susceptibility to infections and dental problems (5). Metabolic inflammation mediated by various pro-inflammatory cytokines (e.g., tumor necrosis factor-α-TNF-α) and chemokines (e.g., chemokine C-C motif ligand-2 and-5) has been recognized to be the leading cause of the onset and advancement of diabetic complications (6). At the initial stage of insulin resistance, macrophage-derived interleukin (IL)-1β can stimulate insulin secretion via promoting β-cell proliferation. However, as insulin resistance persists, the prolonged action of IL-1β stimulates the generation of a wide array of cytokines and chemokines such as IL-6, IL-8 and IL-33. These attract macrophages and immune cells to the pancreatic islet where β-cells are located, further triggering the IL-1β auto-stimulation cycle. The consequence of excessive production of cytotoxic factors from macrophages and IL-1β is the mass reduction of β-cell and its impaired function (7). Moreover, hyperglycemia casts additional inflammatory burden to the pancreatic islet by stimulating the production of pro-inflammatory cytokines that are regulated by the transcription factor, nuclear factor-kappa-B (NF-κB), the central regulator of inflammation. The systemic low-grade inflammation can markedly accelerate tissue injury from pancreatic islet to a wider vascular damage that mediates the progression of diabetic microvascular complications such as diabetic kidney disease, diabetic retinopathy and diabetic neuropathy (8, 9). This highlights the importance of suppressing the diabetes-induced immune-inflammatory responses in treating diabetes and preventing its progression to microvascular complications.
1.1. Diabetic complications
Diabetic complications are classified into macrovascular and microvascular complications (10). The former type comprises coronary artery disease, peripheral arterial disease and stroke; whist the latter is manifested into diabetic eye deterioration (retinopathy), kidney disease (nephropathy) and peripheral nerve damage (neuropathy) caused by changes in the thickness of the capillary basement membrane (11).
1.1.1. Diabetic cardiovascular complications
Diabetes is recognized as an independent risk factor for cardiovascular diseases (CVD) including atherosclerotic coronary heart disease, ischemic heart disease, myocardial infarction and stroke (5). The pathogenesis of CVD involves the development of vascular endothelial dysfunction, atherosclerosis, hypertension, increased oxidative stress and chronic low-grade inflammation. The chronic inflammation and increased oxidative stress resulting from insulin resistance and hyperglycemia in diabetes create an atherothrombotic environment that promotes the development of endothelial dysfunction and atherosclerosis, eventually leading to diabetic cardiovascular complications (DCC). Other factors related to insulin resistance, such as dyslipidemia and hypertension, further elevate the risk of CVD in diabetes (12).
1.1.2. Diabetic nephropathy
Diabetic nephropathy (DN) is considered one of the most common diabetic microvascular complications, prevailing between 30 and 50% of patients with diabetes. It is largely responsible for the end-stage renal failure in patients with diabetes (13). The clinical symptoms feature progressive proteinuria and a noticeable decline in glomerular filtration rate, indicative of deteriorating function of renal nephrons. Pathogenesis of DN involves the elevated glomerular basement membrane thickness, microaneurysm formation, mesangial nodule formation (Kimmelsteil–Wilson bodies), and other changes. Chronic inflammation is identified as the key causative factor for the DN and the advancement of the condition is closely related to a bundle of pro-inflammatory cytokines, such as IL-1, IL-6 and TNF-α (14). The recent research provides new insights in the therapeutic treatment for DN by targeting at inflammatory response and pro-inflammatory cytokines for DN.
1.1.3. Diabetic retinopathy
Diabetic retinopathy (DR) is the primary cause of blindness in patients with diabetes. The DR occurs in around 35% (92.6 million) among diabetic adult patients, of which 28.4 million suffering from vision impairment (15, 16). It involves changes in retinal ganglion cells (RGCs). Patients with prolonged duration of diabetes and poor control of hyperglycemia and hypertension are prone to developing DR. The sustained diabetes-evoked inflammatory responses mediated by pro-inflammatory modulators (IL-1β, TNF-α) majorly contribute to the development of DR, consequently altering the retinal structure and impairing its function. In addition, growth factors including vascular endothelial growth factor (VEGF) and transforming growth factor beta (TGF-β), also play important roles in the development of DR. In general, the mechanistic basis of the development of DR resembles that for DN (17).
1.1.4. Diabetic peripheral neuropathy
Diabetic peripheral neuropathy (DPN), as the most common long-standing diabetic neuropathy, affects the sensory and autonomic nervous system. More than half of the diabetic patients suffers from this complication (18). Symptoms of DPN are often manifested as pain and abnormal heat/cold sensitivity, making patients susceptible to increased risk of burns, injuries, foot ulceration, and the need for amputations (19). The pathogenesis of DPN is complex, primarily involving axonal atrophy, demyelination, blunted regeneration, and loss of neuronal fibers. Hyperglycemia and dyslipidemia in the presence of insulin resistance are the major contributors to DPN’s progression. The injury to peripheral nerves triggers enhanced inflammatory responses via the action of inflammatory cytokines such as TNF-α (20).
In general, the clinically diverse microvascular complications of diabetes share a similar root of their pathogenesis, i.e., all involving hyperglycemia and increased oxidative stress, inflammation, and formation of advanced glycation end products (AGEs).
1.2. Diabetes management
The goal of diabetes management is to achieve optimal glycemic control and prevent or delay the onset and progression of diabetic complications. Dietary and lifestyle modification are recommended approaches for managing diabetes, together with pharmacological interventions including oral hypoglycemic medications (e.g., glipizide, metformin, or acarbose) or ultimately insulin injections (21). Yet, diabetic patients commonly experience issues of taking anti-diabetic medications, such as hypoglycemia, weight gain, headache and nausea and the financial cost (21). Emerging preclinical and clinical evidence has demonstrated notable benefits of inflammation-targeted dietary therapies using natural products in treating hyperglycemia, β-cell dysfunction, and insulin resistance in diabetes (6, 10). Moreover, cohort studies have shown that greater intake of green leafy vegetables was associated with a reduction in risk of T2DM (22, 23). Notably, flavonoids and carotenoids, natural phytochemicals commonly found in edible fruits and vegetables (F&V), have been shown to mediate immune-inflammatory responses and modulate non-cytokine mediators such as reactive oxygen species and nitric oxide (NO), thus potentially protecting against diabetes-related complications (24–27). A large body of research has focused on the preventive effects of flavonoids and carotenoids against the immediate effects of T2DM (28–32), but their protective effects against diabetic complications have remained less studied, with clinical evidence emerging but still limited (33, 34). This review will primarily discuss the therapeutic potential of dietary flavonoids and carotenoids in preventing/treating diabetic complications based on in vivo evidence and examine the possible mechanistic bases involved with a particular focus on the immune-inflammation modulatory effects of those chemicals.
2. Flavonoids and flavonolignans
Flavonoids are widely found as secondary metabolites in F&V, notably berries and citrus fruits, leafy vegetables, onions and beverages like tea and wine (35). Their polyphenolic structure features two benzene rings attached by a short three carbon chain. Flavonoids can be divided into sub-categories including flavonols (e.g., quercetin, kaempferol; Figures 1, 2), flavanols (e.g., epicatechin; Figure 3), flavones (e.g., chrysin, luteolin, and diosmin; Figures 4–7), isoflavones (e.g., genistein; Figure 8), flavanones (e.g., eriodictyol, hesperetin, and naringenin; Figures 9–11), anthocyanins (e.g., pelargonidin; Figure 12) (35). Silymarin is a standardized mixture of different flavonolignans extracted from the seeds of the milk thistle (Silybum marianum (L.) Gaertn.). It comprises a mixture of flavonolignans such as isosilibinin, silychristin, and silydianin (36) (Figure 13). The antidiabetic properties of certain flavonoids and flavonoligans have been widely reported, including hypoglycemic, antioxidant and anti-inflammatory effects and their modulatory role in cell signaling and immune-inflammation responses (37). The names and dietary sources of a few flavonoids that have shown antidiabetic functions in studies are presented in Table 1.
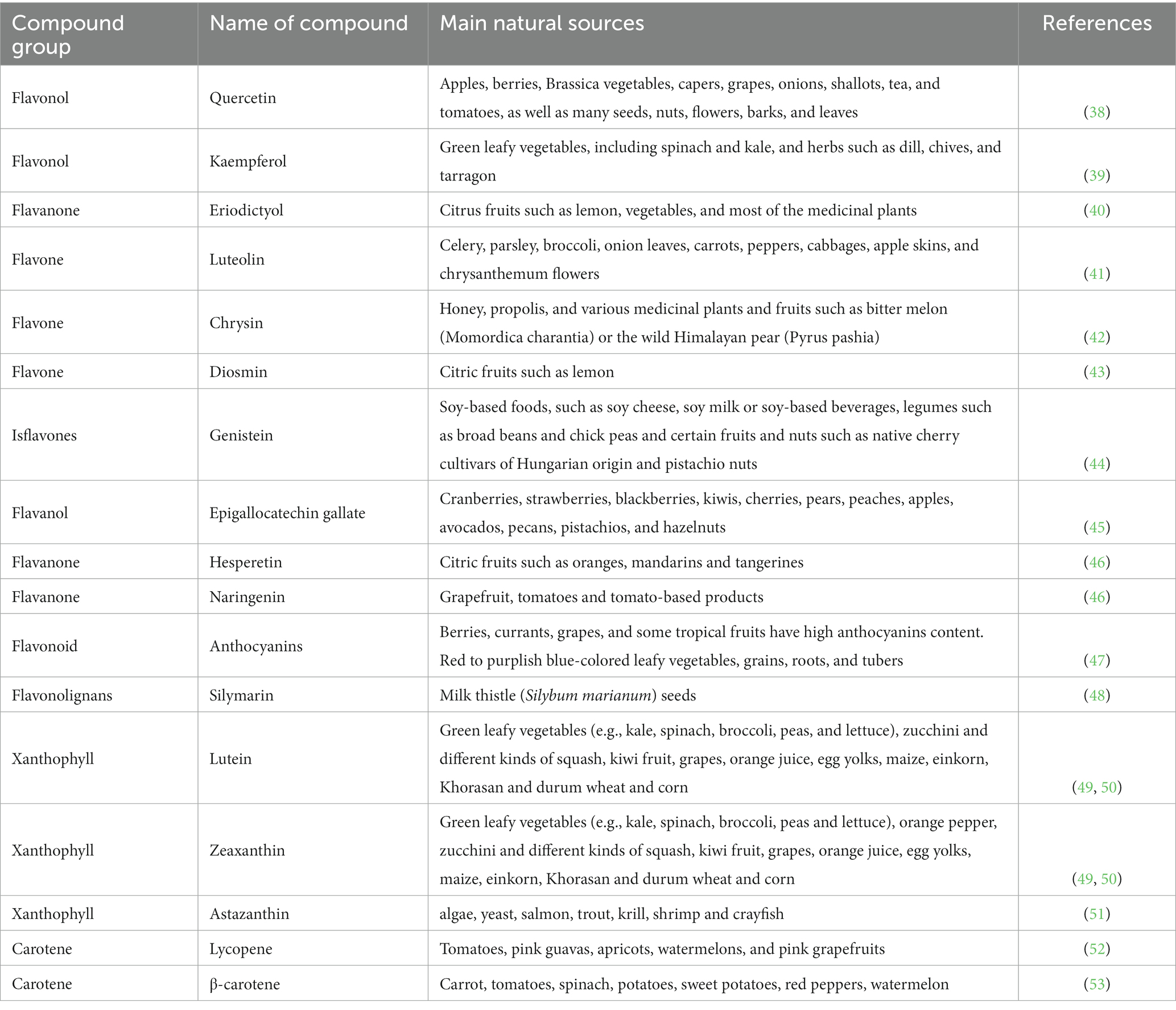
Table 1. Major sources of flavonoids and carotenoids used in the treatment of diabetes and its complications.
3. Carotenoids
Carotenoids are a diverse group of lipid-soluble pigments occurring in a wide array of photosynthetic organisms including plants, algae and cyanobacteria. To date, more than 650 types of carotenoids have been identified which all share the basic chemical structure of eight isoprene units, forming a C40 backbone (54). Carotenoids are subdivided into two types: carotenes, made only of hydrocarbons (e.g., carotenes and lycopene; Figures 14, 15), and xanthophylls (e.g., lutein, zeaxanthin and astaxanthin) which contain one or more oxygen atoms (Figures 16–18). Among the 30–40 of carotenoid metabolites present in human blood, β-carotene, lycopene, lutein, β-cryptoxanthin and zeaxanthin share the bulk (55). The chemical properties of carotenoids, notably their potent antioxidant capacity, have been well studied. Recently, there has been a growing interest in their biological activities in relation to their potential role in prevention of certain chronic diseases such as diabetes and related complications (56–58). Table 1 presents the names and natural sources of a few types of carotenoids with reported antidiabetic potential in animal and in clinical studies.
4. Evidence on protective effects of flavonoids against diabetic complications
4.1. Animal study evidence
The cardioprotective effects of flavonoids have been shown in diabetic animal models using a range of supplementation doses from 2 to 300 mg/kg body weight (bwt) /day with various durations (4–24 weeks; Table 2). Quercetin ameliorated diabetes-induced vasoconstriction and reduced the elevated blood pressure in diabetic rats when dosed at 50 mg/kg bwt daily for 6 weeks (59). The flavonol also inhibited diabetes-induced adventitial leukocyte infiltration, endothelial pyknosis and increased collagen deposition. Immunohistochemical assays indicated that the cardioprotective effects of quercetin could be attributed to its anti-inflammatory effects exhibited via its inhibition of aortic NF-κB and reducing the serum level of both TNF-α and C-reactive protein (CRP). It presented the potential to prevent the onset of DCC through interference with inflammatory pathways especially NF-κB signaling (59). Another flavonol, kaempferol, presented a dose–response effect at an ingestion level of 50 or 150 mg/kg/day for 10 weeks in attenuating hyperglycemia and hyperinsulinemia in streptozotocin (STZ)-induced diabetic rats. It showed potent anti-inflammatory effects by reducing nucleic and cystosolic levels of NF-κB, TNF-α and IL-6. It also altered the insulin signaling defect by inhibiting the phosphorylation of insulin receptor substrate-1 (IRS-1), IκB kinase α (IKKα) and IκB kinase β (IKKβ) (60). Oral ingestion of the isoflavone genistein in STZ-induced diabetic rats, at a higher dosage (300 mg/kg/day) and for a longer period (24 weeks), restored the integrity of their myocardia, accompanied by reducing diabetes-induced cardiac inflammation and oxidative stress via the reduction of TNF-α, CRP and TGF-β1. Furthermore, the results showed a notable amelioration of the ultrastructural degenerative changes in the diabetes-injured cardiac tissues (61). Supplementation with the flavone luteolin provided a similar cardioprotective effect in STZ-induced diabetic mice by preventing cardiac fibrosis, hypertrophy and dysfunction. The heart tissue injury was prevented by luteolin’s modulations of nuclear factor-erythroid two related factor two (Nrf2)-mediated-oxidative stress and NF-κB-mediated inflammatory responses (62). STZ-induced diabetic rats treated with epigallocatechin-3-gallate (ECGC) exhibited a decreased level of Deoxyribonucleic acid (DNA) damage in the myocardium. Histological examinations further indicated the cardioprotective effect of EGCG through preventing diabetes-induced damage, i.e., reducing the cardiac fibrosis in cardiac tissues whilst improving most of the cardiac muscle fibers. Other diabetic phenotypes including hyperglycemia, dyslipidemia and increased oxidative stress were all significantly improved via ECGC’s antihyperglycemic, antidyslipidemic, and antioxidative actions (63). Lastly, STZ-induced diabetic rats, fed with anthocyanins (250 mg/kg/day) for 4 weeks, showed a significant restoration of their deteriorating cardiac functions with a remarkable reduction in their cardiac hypertrophy and fibrosis, compared to the controls (64).
The protective effects against DR were also seen with other types of flavonoids (Table 2). Hesperetin prevented the onset of DR in a STZ-induced diabetic rat model. The supplementation at 100 mg/kg/day for 24 weeks restored levels of the retinal antioxidant peptide glutathione (GSH) close to non-diabetic levels. Furthermore, hesperetin considerably reduced the retinal levels of inflammatory cytokines including TNF-α and IL-1β and hampered the expression of caspase-3, glial fibrillary acidic protein (GFAP) and aquaporin-4 (AQP4) in retinae which play a key role in retinal degeneration, and prevented the photoreceptor degeneration (65). Eriodictyol acted on a different mechanism in its protective role against DR in diabetic rats. The treatment attenuated the degree of retinal inflammation and plasma lipid peroxidation and prevented the breakdown of the Blood-Retinal-Barrier (BRB) in early STZ-induced diabetic rats. Notably, the reduction in retinal TNF-α, Intercellular Adhesion Molecule 1 (ICAM-1), vascular endothelial growth factor (VEGF), and endothelial nitric oxide synthase (eNOS) followed a dose–response manner using ingestion levels of 0.1, 1 and 10 mg/kg/day, with the last dosage being the most effective in DR prevention (25).
The anti-inflammatory activities of flavonoids have also been manifested in their protection against DN (Table 2). In diabetic mice, naringenin, when supplemented at 1 and 2% of their diet for 10 weeks, attenuated DN via its anti-inflammatory and antifibrotic activities. Both treatments significantly decreased the renal level of interleukin (IL)-1β, IL-6, and monocyte chemoattractant protein (MCP)-1, lowered protein kinase C (PKC) activity and suppressed the NF-κB p65 activity, mRNA expression, and protein production in kidney. The higher dose of 2% further decreased renal formation and expression of type IV collagen, fibronectin, and TGF-β1. Both doses increased the deposit of naringenin in the liver and kidney of diabetic mice (66). The findings suggested that naringenin may be helpful for preventing diabetic nephropathy. In a similar experimental setting, diabetic mice fed on high-fat-diet experienced improved renal pathology, abrogated renal dysfunction and oxidative stress upon an intervention with chrysin at 40 mg/kg/day for 16 weeks. Chrysin’s protective effect against DN is specifically mediated through its anti-inflammatory effects in the kidney targeting the TNF-α pathway (67).
4.2. Clinical evidence
Few human intervention studies thus far have shed light on the promising potential of flavonoids in tackling diabetic phenotypes and its complications. Flavonoids used in those studies was either dosed in their single or mixed isolation forms, or consumed as a whole food among diabetic patients with or without complications developed (Table 3). In a dietary intervention among 31 patients with T2DM, a 6-week daily intake of 500 mg of hesperetin significantly decreased the systolic blood pressure (SBP), diastolic blood pressure (DBP), levels of inflammatory markers including TNF-α, IL-6 and high sensitivity CRP (hs-CRP) and increased the serum total antioxidant capacity (TAC). There were no adverse events or side effect reported throughout the hesperidin supplementation. These results indicate hesperetin’s anti-inflammatory potential, and possible role in helping diabetic patients control their blood pressure naturally and safely (68). The mechanistic basis of the observed effects might have been further explained if the analyses of endogenous antioxidant enzymes such as superoxide dismutase (SOD) and GSH and serum NO level were included in the study design. ECGC supplementation at a dosage of 300 mg/day for 8 weeks remarkedly decreased the level of fasting blood glucose (FBG), hs-CRP as well as Body Mass Index (BMI) compared to baseline, among 50 patients with T2DM (69). The anti-inflammatory effect of ECGC was mediated through its inhibition of NF-κB pathway and suppression of the production of chemokines and inflammatory adipokines (74–76). The findings suggested a potential role of ECGC in improving glycemic control and inflammation status in T2DM. Unfortunately, glycosylated hemoglobin (HbA1c) levels were not considered in the analysis and only a relatively small cohort was recruited, i.e., 50 subjects in the intervention group. Care should be taken in order not to overinterpret the results of this study. The cardioprotective effect of flavonoids in diabetes was also observed when dosed as a whole food to male patients with T2DM. Upon daily intake of 22 g of freeze-dried blueberries (equivalent to one cup of fresh blueberries) for 8 weeks, in addition to habitual diet, there was a notable improvement in the cardiometabolic parameters of the 52 participants, shown as the reduced serum levels of HbA1c and fructosamine, total cholesterol (TC), low-density-lipoprotein-C and triglycerides. However, other parameters including FBG, insulin, CRP and high-density-lipoprotein cholesterol (HDL-C) and blood pressure were not affected by intervention (70). This study was one of the very first clinical studies examining the effect of freeze-dried blueberries in treating diabetic conditions. Again, sample size was a limiting factor of this trial that may have hindered the detection of differences in some key biomarkers between the intervention and placebo groups. Also, the fiber intake differed between the two groups, with the freeze-dried blueberry intervention containing 5.2 g of fiber and the placebo containing 0.3 g. Given an increased fiber intake can independently improve glycemic response (77, 78), further consideration is needed in the study design to identify the key influencer (i.e., either anthocyanins and phenolics from blueberries, or fiber content) on the glycemic control. Also, the overall efficacy observed could be better interpreted if data on the bioavailability of anthocyanins and other phenolics in blueberries were made available, as the presence of DN largely among participants at the start of the trial might have affected the bioavailability and functionality of the polyphenols consumed (79).
Silymarin exhibited positive effect in preventing the progression of DN among 30 patients with T2DM and macroalbuminuria (urinary albumin excretion >300 mg/24 h) at a dosage level of 140 mg of silymarin taken thrice daily for 3 months. The intervention resulted in a higher decrement of urinary albumin-creatinine ratio (UACR) from baseline level compared to that of the placebo group, and also a significant reduction in the urinary levels of TNF-α and both urinary and plasma levels of malondialdehyde (MDA)—a marker of lipid peroxidation compared to placebo group (71). The results suggested silymarin be used as a novel agent to treat DN based on its antidiabetic and antioxidant potentials. Showing the similar renal-protective effects, the daily ingestion of a mixture of flavonoids (i.e., flavones/flavonols, flavanones/dihydroflavonols, and phenolic compounds) contained in Iranian propolis capsules at the level of 1,000 mg for 90 days majorly improved the diabetic phenotype, elevated the HDL-C and prevented renal dysfunction in 50 diabetic patients. The beneficial effects were observed through the reduction in postprandial blood glucose, serum insulin, insulin resistance, and inflammatory cytokines at the end of intervention (72). The effects of propolis may be ascribed to the synergistic actions of multiple compounds instead of isolated individual ones, however, the effective components and their doses, within the capsules, were not identified in the study (80). In another clinical trial using a mixture of flavonoids containing 300 mg of diosmin, 15 mg of Centella asiatica and 160 mg of Melilotus officinalis, the supplementation among 35 patients with T2DM and diabetic cystoid macular edema (CME) but without macular thickening remarkably restored the retinal sensitivity at months 12, 24, and 36 compared to the untreated group. But no differences in HbAc1, blood pressure, microalbuminuria, visual acuity, mean central retinal thickness, and stability of fixation were found between test and placebo groups during follow-up. No side effects from the intervention were reported, suggesting the safety and potential efficacy of using the compound mix as a natural agent for preventing DR (73).
5. Evidence on protective effects of carotenoids against diabetic complications
5.1. Animal study evidence
Lutein, zeaxanthin, lycopene and astaxanthin are the most-studied carotenoids in diabetic animal models for their putative therapeutic effects in preventing or treating diabetic complications, commonly DR (Table 4). In a mice model with an early stage of T2DM, the supplementation of 1% (kcal) wolfberry rich in lutein and zeaxanthin (a.k.a. Goji berry—Lycium barbarum L.) to the background diet for 8 weeks ameliorated mouse retinal abnormality via restoring the thickness of the entire retina, the structure of the inner nuclear layer and photoreceptor layer, and the integrity of retinal pigment epithelia (RPE), and the ganglion cell number in retina (81). The study further investigated the mechanism of wolfberry’s action on retinal structure restoration in diabetic mice by analyzing the retinal cell lysates of those mice treated with wolfberry supplements. The findings revealed that the wolfberry supplement downregulated the expression of endoplasmic reticulum (ER) stress biomarkers including binding immunoglobulin protein (BiP), protein kinase RNA-like ER kinase (PERK), activating transcription factor 6 (ATF6), and caspase-12. In contrast, the supplement restored the activities of AMP-activated protein kinase (AMPK) that regulates metabolic control in dedicated tissues such as the liver, muscle and fat (675). Similar effects were also exerted on, thioredoxin and manganese superoxide dismutase (MnSOD) as endogenous antioxidants (89, 90), and Forkhead O transcription factor 3α (FOXO3α). FOXO3 α protects cells from oxidative stress in diabetes upon activation (91, 92). ER stress majorly contributes to the cellular oxidative damage in the diabetic retina and eventually retinal cell apoptosis as a result of hyperglycemia and insulin resistance (93). Therefore, attenuating ER stress through modulating the expression of its sensor proteins/biomarkers, as the effect exerted by wolfberry supplement in this study, has been considered as one of the novel targets for diabetic therapy (94). Regarding the bioactive components in wolfberry that may have contributed to its retinal protective effect in the study, it was further confirmed through an additional in vitro experiment in the same study that the retinal-protective effect of wolfberry was at least partially due to its lutein and/or zeaxanthin content that showed to attenuate ER stress in ARPE-19 cells exposed to a high glucose challenge (81). In another study using diabetic mice, lutein on its own showed adjuvant effect in treating diabetes-induced oxidative stress in both retina and hippocampus (82). Hippocampus as part of the central nervous system is specifically susceptible to prolonged-hyperglycemia-induced alterations (95), i.e., apoptosis-induced neuronal loss leading to cognitive impairment after 8 months of diabetes, as reported in diabetic rat models (96). This study confirmed an initial impairment of hippocampus in untreated diabetic rats based on the positive correlation observed between the HbA1c values and hippocampal dysfunction. Also, retinal dysfunction was detected after 15 days of diabetes in rats. Lutein’s intragastrical ingestion at 0.2 mg/kg/day for 14 days restored the adverse changes in both retina and hippocampus of the diabetic rats. The protective effects were related to lutein’s antioxidant and anti-inflammatory activities, exhibited via its prevention of increases in oxidative stress (measured by the MDA and GSH concentrations and glutathione peroxidase (GPx) activity) and NF-κB activity in both diabetic retina and hippocampus (82). Zeaxanthin, on the other hand, also demonstrated the potential of preventing the development of retinopathy in diabetic rats when supplemented alone at 0.02% or 0.1% (w/w) for 8 weeks. It was found to share the similar mechanistic basis to that of lutein in its protective role (83).
The efficacy of lycopene in preventing DN has been tested in several diabetic rat models, using a daily dose range between 5 and 20 mg/kg (Table 4). After an oral or intragastrical feeding for 8 or10 weeks in diabetic rats, lycopene prevented diabetes-induced renal lesions and improved renal function via attenuating oxidative stress by increasing the activities of endogenous antioxidants such as SOD and GPx and decreasing MDA and oxidized (ox)-LDL, and inhibiting NF-κB signal pathway. In addition, lycopene exhibited anti-hyperglycemia and anti-dyslipidemia properties by decreasing the FBG and modulating plasma levels of TC, LDL-C, HDL-C and triglycerides (84, 85). The findings support lycopene’s protective role against diabetic progression and further complications through various mechanisms.
Astaxanthin has demonstrated both protective potentials against DN and DR in diabetic db/db mice as a model of T2DM (Table 4). An oral dosage of 0.02% (w/w) astaxanthin for 12 weeks inhibited the progression and acceleration of diabetic nephropathy in diabetic db/db mice and also lowered blood glucose level. The antioxidative activity of astaxanthin attenuated the renal oxidative stress and prevented renal cell damage (86). The retinal protective effect of astaxanthin was further confirmed in another study using diabetic db/db mice with DR developed. The treatment of either 25 or 50 mg/kg/day for 8 weeks inhibited the apoptosis of retinal ganglion cells (RGCs) and improved the levels of oxidative stress markers in the retinal tissues of db/db mice (87). Since apoptosis of RGCs is the key driver for DR, astaxanthin may serve as a potential antioxidant treatment for this complication.
Astaxanthin and lutein also, respectively, showed neuroprotective effect in the retina of diabetic rats upon a supplementation of either astaxanthin (0.6 or 3 mg/kg) or lutein (0.5 mg/kg) daily for 6 weeks (Table 4). The xanthophyll carotenoids reduced ocular oxidative stress and inflammation mediated by downregulating NF-κB activity (88).
5.2. Clinical evidence
A few human intervention studies have been emerging recently in exploring the safety and efficacy of using carotenoids in either an oral supplement or a food form in preventing or treating diabetic complications mainly DR, DCC, and DPN (Table 5). In a case–control study involving 30 patients with non-proliferative diabetic retinopathy (NDR), a 3-month dose of mixed lutein (6 mg/d) and zeaxanthin (0.5 mg/d) supplement improved the visual acuity, contrast sensitivity (CS) and macular edema of the DR patients, concomitantly with increased serum levels of lutein and zeaxanthin, suggesting that the mixed xanthophylls possess a therapeutic potential in treating NDR. Regarding the safety of consumption, one patient reported having headache after the supplement administration, which was possibly due to allergy to the compound mixture, as explained by the researchers, but the exact reason was unknown (97). The effects of lutein and zeaxanthin together in preventing DR was also examined in a 2-year intervention study among 60 patients with T2DM. A supplement complex containing lutein (10 mg), zeaxanthin (2 mg) and meso-zeaxanthin (10 mg) resulted in a rise in the central foveal thickness and the multifocal electroretinography revealed increased retinal response density within the central 13° surrounding the fovea (rings 1–3) at 2 years of the supplementation (98). The results establish a sound foundation in building future trials to assess the long-term efficacy of using carotenoid supplementation in improving the visual function of type 2 diabetic patients. Similarly, another study using the supplement of lutein and zeaxanthin together with antioxidants (vitamin Cand mixed tocotrienols/tocopherols) and selected botanical extracts delivered in a capsule form significantly improved the visual function and the macular pigment optical density (MPOD) and ameliorated the peripheral neuropathy and the hsCRP level in patients with diabetes, both with and without retinopathy. The supplement also optimized the lipid profile of the patients by lowering LDL-C and triglycerides, with no effect on glycemic control (99). Although the study did not assess the bio-availability of individual compounds contained in the mixture upon ingestion, the increment of MPOD readings from baseline measures indicated the retinal uptake of lutein and zeaxanthin. Such multi-component formula may exert synergistic or inhibitory constituent effects, yet without a clear picture of the constituent bio-availabilities, the interpretation of the mechanistic basis is limited. The study was single-centered with a short duration, which warrants future research employing a wider patient community and longer evaluation time. In a comparable study setting, lutein was supplemented alone to 15 patients with NDR at 10 mg/day for 36 weeks. The treatment elevated the CS at four spatial frequencies, with the increment significantly exceeding that of the placebo group at 3 cycles/degree. Although not significant, a slight increase in glare sensitivity (GS) in the lutein group was also observed between baseline and week 36 (100). Lutein supplement might benefit as an adjunct therapy in preventing vision deterioration and inhibit advancement of DR in diabetic patients, yet such notion needs to be further confirmed in a larger-scaled clinical trial.
Echoing the animal study findings, lycopene administered in an oral supplement or whole-food form showed cardioprotective effect among patients with T2DM (Table 5). A 10 mg/day supplementation for 2 months attenuated T cell-dependent adaptive (pro-atherogenic) immune response, and prevented the oxidized-LDL uptake by macrophage and foam cell formation, accompanied with increased serum lycopene levels and antioxidant capacity among 16 patients with T2DM (101). Comparatively, when lycopene taken as 250 ml tomato juice twice daily among 15 patients with T2DM, the intrinsic resistance of LDL to oxidation increased almost as effectively as supplementation with a high dose of vitamin E and the plasma levels of CRP in patients also declined, together with an elevation in plasma lycopene levels (102). The results are suggestive on the preventive role of lycopene against long-term diabetic complications, notably CVD. β-Carotene ameliorated glucose intolerance and improved the plasma lipid profile and NO and GSH levels in 51 patients with T2DM when consumed in a fortified synbiotic food containing 0.05 g β-carotene (103). Although the results favor a conclusion on β-carotene’s antidiabetic role, its cardioprotective effect was not confirmed in a large randomized controlled trial (RCT) (104). As part of the α-Tocopherol, β-Carotene Cancer Prevention Study, 29,133 middle-aged male smokers received either vitamin E (50 mg/day) or β-carotene (20 mg/day), or both, or placebo for a median of 6.1 years. The cohort (1700 men) had ~5% being diagnosed withtT2DM, among whom 662 had the first-onset macrovascular complications. Neither intervention made any changes in the risk of macrovascular complication or total mortality. It was concluded that β-carotene or α-tocopherol supplementation has no protective effect on macrovascular outcomes or total mortality of diabetic male smokers (104). However, the study result cannot be generalized to women and non-smokers, and the specificity of its design toward diabetic complication prevention is debatable, as the study was primarily targeted at examining the effects of those antioxidants toward lung cancer (104), hence influencing the validity/suitability of the supplement’s dosage, timing and duration in treating diabetes. Contrarily, carotenoids, when taken in a whole food, did present protective effects against the risk of developing CVD among patients with T2DM. Beta-and alpha-carotenes are the predominant forms for carotenoids found in banana fruit (105). In an intervention study, 21 patients with T2DM took 40 g of green (unripe) banana biomass daily for 6 months experienced considerable changes in CVD risk factors, including improved blood lipid profile and glucose tolerance level as well as enhanced protection of the LDL particles against oxidation mediated by an increased carotenoids content in those particles (105). The effective protection against LDL-modifications implies a decreased risk of developing CVD. The glycemic control and anti-dyslipidemia functions of unripe banana were also likely to be partly attributed to its resistance starch content, which has exhibited similar antidiabetic effects upon consumption in both prediabetic and diabetic patients (106). In addition, no adverse events such as diarrhea, constipation and abdominal bloating were reported throughout the intervention, indicating the high tolerability of green banana biomass (38). The efficacy and safety of the green-banana biomass encourage its use as potential therapeutics for patients with T2DM.
6. Discrepancy between outcomes of animal experiments and human trials
Recent meta-analyses of prospective cohort studies have confirmed that intake of flavonoid-rich foods is associated with a lower risk of T2DM. In the studies, intake of flavonoids was calculated using food frequency questionnaires and data from the United States Department of Agriculture1 or from the European Phenol-Explorer Database.2 A significant correlation was found between the intake of anthocyanidins, flavan-3-ols, flavonols, and isoflavones, however, no significant association was observed with respect to either flavanones or flavones (29, 107–109).
Though the cohort studies indicate a beneficial effect of flavonoids, the mechanism of action has remained elusive. Initial studies pointed at the antioxidant activities of flavonoids as a potential mechanism to prevent diabetic complications caused by chronic hyperglycemia. However, the poor bioavailability of flavonoids rarely makes the levels reached in the blood serum or in systemic circulation that are sufficient to give an in vivo antioxidant effect. Admittedly, flavonoids may trigger antioxidant signaling pathways and thus stimulate production of endogenous antioxidants.
An alternative mechanism that may explain the preventive effect of flavonoids against the onset of diabetes may be that they act as α-glucosidase inhibitors in the digestive tract, much like the prescription drug acarbose. Thus, dietary flavonoids may inhibit the absorption of carbohydrates from the small intestine, resulting in lowered blood sugar levels (110, 111). Flavonoids in the digestive tract affect, or alter the composition of the resident gut microflora. The microbiota-gut-brain axis is drawing increasing attention as an important system that connects nerve, hormone and immune signals, and thus regulates human metabolism (28, 111). At present, the role of gut microflora in prevention of diabetes is still a matter of speculation.
Meta-analysis of cross-sectional studies and randomized controlled trials linking serum levels of total carotenoids and vitamin A with metabolic syndrome, showed an inverse association. This association was strongest for β-carotene, followed by α-carotene and β-crypotoxanthin. No significant association was detected between retinol and metabolic syndrome (112).
If medicinal claims are made for dietary phytochemicals, e.g., a role of flavonoids or carotenoids in the prevention or management of diabetes, then the weight of evidence that is needed to claim efficacy ought to be similar to that required in classical pharmacology. However, in nutrition studies it is generally common to ascribe biological activity to complex mixtures or to synergism. That approach would be undesirable in drug design and classical pharmacology and is also not conducive to the elucidation of an unambiguous mechanism of action. Whereas some in vivo animal studies have focused on single flavonoids or carotenoids, very few human intervention studies looked at the effect of single compounds (human cohort studies do not consider single compounds at all). This may explain why proposed mechanisms of action developed from animal studies have never been unambiguously confirmed in human clinical trials.
7. Conclusion and future perspectives
The current preclinical and clinical evidence largely supports the therapeutic roles of the two natural compound groups (flavonoids and carotenoids) in preventing the advancement of diabetic conditions and the onset and progression of diabetic complications, mainly DCC, DN, and DR. Some evidence showed carotenoids’ preventive effects against DPN, but not for flavonoids in this review. Notably, carotenoids’ protective effects are more oriented toward DR. Their modes of therapeutic actions are pleiotropic involving alleviating hyperglycemia, insulin resistance and dyslipidemia, attenuating oxidative stress and metabolic inflammation, elevating insulin sensitivity and antioxidant capacity in tissues and organs, as well as combating key risk factors of complications such as hypertension (for DCC) and increased apoptosis of RGCs (for DR). Among all, the anti-inflammatory and antioxidant properties of flavonoids and carotenoids form their principal mechanistic basis in treating diabetes and preventing/ameliorating its complications (Figure 19), given the pathogenic mechanisms driving chronic diabetic complications primarily involve metabolic inflammation and oxidative stress (113).
From the in vivo studies reviewed, there is a clear discrepancy in the level of dosage and length of feeding duration applied in all experimental settings including human interventions. Also, some beneficial effects may have been derived from synergistic actions of multiple phytochemical compounds administered instead of single ones. Most of the clinical trials to date studied the effect of concentrated phytochemical extracts taken as a supplement rather than that of whole foods rich in those phytochemicals. Very few investigated the bioavailability of the test compounds upon ingestion under diabetic conditions. Hence, the interactions of diabetes (specially hyperglycemia) and its complications with the bioavailability and bioactivity of those phytochemicals are not clearly understood, which limits the extension of knowledge of the mechanisms underlying their protective effects. The existence of other dietary components such as starch, fiber and alkaloids may enhance the antidiabetic potential of those phytochemicals, e.g., flavonoids (114), yet, current clinical trials tend not to consider this aspect in the study design. Also, the present clinical evidence focuses on the short-term effect rather than long term, the latter of which more fits into the development pattern of chronic diabetic complications. Therefore, appropriate and well-designed clinical trials are necessary to identify pharmacologically active dietary phytochemicals and elucidate their mechanism of action in treating diabetes-related complications when dosed as whole foods and on a long-term basis.
In summary, these naturally occurring phytochemicals hold great potential for further development into novel drugs/therapies for treating diabetes and its complications. Yet, future long-term RCTs with bioavailability investigations are needed to identify their effective dose and duration, and safety and tolerability at various doses consumed among patients at different disease stages before confident clinical applications.
Author contributions
All authors listed have made a substantial, direct, and intellectual contribution to the work and approved it for publication.
Conflict of interest
The authors declare that the research was conducted in the absence of any commercial or financial relationships that could be construed as a potential conflict of interest.
Publisher’s note
All claims expressed in this article are solely those of the authors and do not necessarily represent those of their affiliated organizations, or those of the publisher, the editors and the reviewers. Any product that may be evaluated in this article, or claim that may be made by its manufacturer, is not guaranteed or endorsed by the publisher.
Footnotes
References
1. Craig, ME, Hattersley, A, and Donaghue, KC. Definition, epidemiology and classification of diabetes in children and adolescents. Pediatr Diabetes. (2009) 10:3S–12S. doi: 10.1111/j.1399-5448.2009.00568
2. Saeedi, P, Petersohn, I, Salpea, P, Malanda, B, Karuranga, S, Unwin, N, et al. Global and regional diabetes prevalence estimates for 2019 and projections for 2030 and 2045: results from the international diabetes federation diabetes atlas, 9(th) edition. Diabetes Res Clin Pract. (2019) 157:107843. doi: 10.1016/j.diabres.2019.107843
3. Williams, R, Karuranga, S, Malanda, B, Saeedi, P, Basit, A, Besancon, S, et al. Global and regional estimates and projections of diabetes-related health expenditure: results from the international diabetes federation diabetes atlas, 9th edition. Diabetes Res Clin Pract. (2020) 162:108072. doi: 10.1016/j.diabres.2020.108072
4. Irwin, S. Chapter 10-primary prevention and risk reduction for cardiovascular/pulmonary disorders—preferred practice pattern 6A In: S Irwin and JS Tecklin, editors. Cardiopulmonary Physical Therapy. 4th ed. Saint Louis: Mosby (2004). 253–69.
5. Grundy, SM, Benjamin, IJ, Burke, GL, Chait, A, Eckel, RH, Howard, BV, et al. Diabetes and cardiovascular disease: a statement for healthcare professionals from the American Heart Association. Circulation. (1999) 100:1134–46. doi: 10.1161/01.cir.100.10.1134
6. Kong, M, Xie, K, Lv, M, Li, J, Yao, J, Yan, K, et al. Anti-inflammatory phytochemicals for the treatment of diabetes and its complications: lessons learned and future promise. Biomed Pharmacother. (2021) 133:110975. doi: 10.1016/j.biopha.2020.110975
7. Boni-Schnetzler, M, Thorne, J, Parnaud, G, Marselli, L, Ehses, JA, Kerr-Conte, J, et al. Increased interleukin (IL)-1beta messenger ribonucleic acid expression in beta-cells of individuals with type 2 diabetes and regulation of IL-1beta in human islets by glucose and autostimulation. J Clin Endocrinol Metab. (2008) 93:4065–74. doi: 10.1210/jc.2008-0396
8. Forbes, JM, Coughlan, MT, and Cooper, ME. Oxidative stress as a major culprit in kidney disease in diabetes. Diabetes. (2008) 57:1446–54. doi: 10.2337/db08-0057
9. Aiello, LP, Bursell, SE, Clermont, A, Duh, E, Ishii, H, Takagi, C, et al. Vascular endothelial growth factor-induced retinal permeability is mediated by protein kinase C in vivo and suppressed by an orally effective beta-isoform-selective inhibitor. Diabetes. (1997) 46:1473–80. doi: 10.2337/diab.46.9.1473
10. Pollack, RM, Donath, MY, LeRoith, D, and Leibowitz, G. Anti-inflammatory agents in the treatment of diabetes and its vascular complications. Diabetes Care. (2016) 39:S244–52. doi: 10.2337/dcS15-3015
11. Caro-Ordieres, T, Marin-Royo, G, Opazo-Rios, L, Jimenez-Castilla, L, Moreno, JA, Gomez-Guerrero, C, et al. The coming age of flavonoids in the treatment of diabetic complications. J Clin Med. (2020) 9:10. doi: 10.3390/jcm9020346
12. Fowler, MJ. Microvascular and macrovascular complications of diabetes. Clin Diabetes. (2011) 29:116–22. doi: 10.2337/diaclin.29.3.116
13. Faselis, C, Katsimardou, A, Imprialos, K, Deligkaris, P, Kallistratos, M, and Dimitriadis, K. Microvascular complications of type 2 diabetes mellitus. Curr Vasc Pharmacol. (2020) 18:117–24. doi: 10.2174/1570161117666190502103733
14. Selby, NM, and Taal, MW. An updated overview of diabetic nephropathy: diagnosis, prognosis, treatment goals and latest guidelines. Diabetes Obes Metab. (2020) 22:3–15. doi: 10.1111/dom.14007
15. Yau, JW, Rogers, SL, Kawasaki, R, Lamoureux, EL, Kowalski, JW, Bek, T, et al. Global prevalence and major risk factors of diabetic retinopathy. Diabetes Care. (2012) 35:556–64. doi: 10.2337/dc11-1909
16. Ting, DS, Cheung, GC, and Wong, TY. Diabetic retinopathy: global prevalence, major risk factors, screening practices and public health challenges: a review. Clin Exp Ophthalmol. (2016) 44:260–77. doi: 10.1111/ceo.12696
17. Chistiakov, DA. Diabetic retinopathy: pathogenic mechanisms and current treatments. Diabetes Metab Syndr. (2011) 5:165–72. doi: 10.1016/j.dsx.2012.02.025
18. Pop-Busui, R, Boulton, AJ, Feldman, EL, Bril, V, Freeman, R, Malik, RA, et al. Diabetic neuropathy: a position statement by the American Diabetes Association. Diabetes Care. (2017) 40:136–54. doi: 10.2337/dc16-2042
19. Kuhad, A, Sharma, S, and Chopra, K. Lycopene attenuates thermal hyperalgesia in a diabetic mouse model of neuropathic pain. Eur J Pain. (2008) 12:624–32. doi: 10.1016/j.ejpain.2007.10.008
20. Farmer, KL, Li, C, and Dobrowsky, RT. Diabetic peripheral neuropathy: should a chaperone accompany our therapeutic approach? Pharmacol Rev. (2012) 64:880–900. doi: 10.1124/pr.111.005314
21. Chaudhury, A, Duvoor, C, Reddy Dendi, VS, Kraleti, S, Chada, A, Ravilla, R, et al. Clinical review of antidiabetic drugs: implications for type 2 diabetes mellitus management. Front Endocrinol (Lausanne). (2017) 8:6. doi: 10.3389/fendo.2017.00006
22. Carter, P, Gray, LJ, Troughton, J, Khunti, K, and Davies, MJ. Fruit and vegetable intake and incidence of type 2 diabetes mellitus: systematic review and meta-analysis. BMJ. (2010) 341:c4229. doi: 10.1136/bmj.c4229
23. Cooper, AJ, Forouhi, NG, Ye, Z, Buijsse, B, Arriola, L, Balkau, B, et al. Fruit and vegetable intake and type 2 diabetes: EPIC-InterAct prospective study and meta-analysis. Eur J Clin Nutr. (2012) 66:1082–92. doi: 10.1038/ejcn.2012.85
24. Shin, SA, Joo, BJ, Lee, JS, Ryu, G, Han, M, Kim, WY, et al. Phytochemicals as anti-inflammatory agents in animal models of prevalent inflammatory diseases. Molecules. (2020) 25:5932. doi: 10.3390/molecules25245932
25. Bucolo, C, Leggio, GM, Drago, F, and Salomone, S. Eriodictyol prevents early retinal and plasma abnormalities in streptozotocin-induced diabetic rats. Biochem Pharmacol. (2012) 84:88–92. doi: 10.1016/j.bcp.2012.03.019
26. Ozawa, Y, Sasaki, M, Takahashi, N, Kamoshita, M, Miyake, S, and Tsubota, K. Neuroprotective effects of lutein in the retina. Curr Pharm Des. (2012) 18:51–6. doi: 10.2174/138161212798919101
27. Calabró, V, Litterio, MC, Fraga, CG, Galleano, M, and Piotrkowski, B. Effects of quercetin on heart nitric oxide metabolism in l-NAME treated rats. Arch Biochem Biophys. (2018) 647:47–53. doi: 10.1016/j.abb.2018.03.041
28. Han, S, Luo, Y, Hu, Z, Qin, D, and Luo, F. Targeting gut microbiota in type 2 diabetes mellitus: potential roles of dietary flavonoids. Food Biosci. (2022) 45:101500. doi: 10.1016/j.fbio.2021.101500
29. Xu, H, Luo, J, Huang, J, and Wen, Q. Flavonoids intake and risk of type 2 diabetes mellitus: a meta-analysis of prospective cohort studies. Medicine (Baltimore). (2018) 97:e0686. doi: 10.1097/MD.0000000000010686
30. Sluijs, I, Cadier, E, Beulens, JW, Van Der ADL, SAM, and van der Schouw, YT. Dietary intake of carotenoids and risk of type 2 diabetes. Nutr Metab Cardiovasc Dis. (2015) 25:376–81. doi: 10.1016/j.numecd
31. Similä, ME, Kontto, JP, Virtamo, J, Hätönen, KA, Valsta, LM, Sundvall, J, et al. Insulin-like growth factor I, binding proteins −1 and −3, risk of type 2 diabetes and macronutrient intakes in men. Br J Nutr. (2019) 121:938–44. doi: 10.1017/S0007114519000321
32. Sluijs, I, Beulens, JW, Grobbee, DE, and van der Schouw, YT. Dietary carotenoid intake is associated with lower prevalence of metabolic syndrome in middle-aged and elderly men. J Nutr. (2009) 139:987–92. doi: 10.3945/jn.108.101451
33. Cao, H, Ou, J, Chen, L, Zhang, Y, Szkudelski, T, Delmas, D, et al. Dietary polyphenols and type 2 diabetes: human study and clinical trial. Crit Rev Food Sci Nutr. (2019) 59:3371–9. doi: 10.1080/10408398.2018.1492900
34. Jin, Y, and Arroo, RRJ. Application of dietary supplements in the prevention of type 2 diabetes-related cardiovascular complications. Phytochem Rev. (2021) 20:181–209. doi: 10.1007/s11101-020-09710-8
35. Hollman, PC, and Katan, MB. Dietary flavonoids: intake, health effects and bioavailability. Food Chem Toxicol. (1999) 37:937–42. doi: 10.1016/s0278-6915(99)00079-4
36. Katiyar, SK, Meleth, S, and Sharma, SD. Silymarin, a flavonoid from milk thistle (Silybum marianum L.), inhibits UV-induced oxidative stress through targeting infiltrating CD11b+ cells in mouse skin. Photochem Photobiol. (2008) 84:266–71. doi: 10.1111/j.1751-1097.2007.00241.x
37. Waheed Janabi, AH, Kamboh, AA, Saeed, M, Xiaoyu, L, BiBi, J, Majeed, F, et al. Flavonoid-rich foods (FRF): a promising nutraceutical approach against lifespan-shortening diseases. Iran J Basic Med Sci. (2020) 23:140–53. doi: 10.22038/IJBMS.2019.35125.8353
38. Lotfollahi, Z, Mello, APQ, Costa, ES, et al. Green-banana biomass consumption by diabetic patients improves plasma low-density lipoprotein particle functionality. Sci Rep. (2020) 10:12269. doi: 10.1038/s41598-020-69288-1
39. Dabeek, WM, and Marra, MV. Dietary Quercetin and Kaempferol: bioavailability and potential cardiovascular-related bioactivity in humans. Nutrients. (2019) 11:2288. doi: 10.3390/nu11102288
40. He, P, Yan, S, Wen, X, Zhang, S, Liu, Z, Liu, X, et al. Eriodictyol alleviates lipopolysaccharide-triggered oxidative stress and synaptic dysfunctions in BV-2 microglial cells and mouse brain. J Cell Biochem. (2019) 120:14756–70. doi: 10.1002/jcb.28736
41. Neuhouser, ML. Dietary flavonoids and cancer risk: evidence from human population studies. Nutr Cancer. (2004) 50:1–7. doi: 10.1207/s15327914nc5001_1
42. Stompor-Gorący, M, Bajek-Bil, A, and Machaczka, M. Chrysin: perspectives on contemporary status and future possibilities as pro-health agent. Nutrients. (2021) 13:2038. doi: 10.3390/nu13062038
43. Benavente-García, O, and Castillo, J. Update on uses and properties of citrus flavonoids: new findings in anticancer, cardiovascular, and anti-inflammatory activity. J Agric Food Chem. (2008) 56:6185–205. doi: 10.1021/jf8006568
44. Spagnuolo, C, Russo, GL, Orhan, IE, Habtemariam, S, Daglia, M, Sureda, A, et al. Genistein and cancer: current status, challenges, and future directions. Adv Nutr. (2015) 6:408–19. doi: 10.3945/an.114.008052
45. James, KD, Forester, SC, and Lambert, JD. Dietary pretreatment with green tea polyphenol, (−)-epigallocatechin-3-gallate reduces the bioavailability and hepatotoxicity of subsequent oral bolus doses of (−)-epigallocatechin-3-gallate. Food Chem Toxicol. (2015) 76:103–8. doi: 10.1016/j.fct.2014.12.009
46. Erlund, I. Review of the flavonoids quercetin, hesperetin, and naringenin. Dietary sources, bioactivities, bioavailability, and epidemiology. Nutr Res. (2004) 24:851–74. doi: 10.1016/j.nutres.2004.07.005
47. Khoo, HE, Azlan, A, Tang, ST, and Lim, SM. Anthocyanidins and anthocyanins: colored pigments as food, pharmaceutical ingredients, and the potential health benefits. Food Nutr Res. (2017) 61:1361779. doi: 10.1080/16546628.2017.1361779
48. Choe, U, Whent, M, Luo, Y, and Yu, L. Total phenolic content, free radical scavenging capacity, and anti-cancer activity of silymarin. J Food Bioact. (2020) 10:53–63. doi: 10.31665/JFB.2020.10226
49. El-SM, A-A, Akhtar, H, Zaheer, K, and Ali, R. Dietary sources of lutein and zeaxanthin carotenoids and their role in eye health. Nutrients. (2013) 5:1169–85. doi: 10.3390/nu5041169
50. Sommerburg, O, Keunen, JE, Bird, AC, and van Kuijk, FJ. Fruits and vegetables that are sources for lutein and zeaxanthin: the macular pigment in human eyes. Br J Ophthalmol. (1998) 82:907–10. doi: 10.1136/bjo.82.8.907
51. Ambati, RR, Phang, SM, Ravi, S, and Aswathanarayana, RG. Astaxanthin: sources, extraction, stability, biological activities and its commercial applications--a review. Mar Drugs. (2014) 12:128–52. doi: 10.3390/md12010128
52. Khan, UM, Sevindik, M, Zarrabi, A, Nami, M, Ozdemir, B, Kaplan, DN, et al. Lycopene: food sources, biological activities, and human health benefits. Oxidative Med Cell Longev. (2021) 2021:2713511–20. doi: 10.1155/2021/2713511
53. Akram, S, Mushtaq, M, and Waheed, A. Chapter 1-β-carotene: beyond provitamin a In: M Mushtaq and F Anwar, editors. A Centum of Valuable Plant Bioactives : Academic Press (2021). 1–31.
54. Fathalipour, M, Fathalipour, H, Safa, O, Nowrouzi-Sohrabi, P, Mirkhani, H, and Hassanipour, S. The therapeutic role of carotenoids in diabetic retinopathy: a systematic review. Diabetes Metab Syndr Obes. (2020) 13:2347–58. doi: 10.2147/DMSO.S255783
55. Milani, A, Basirnejad, M, Shahbazi, S, and Bolhassani, A. Carotenoids: biochemistry, pharmacology and treatment. Br J Pharmacol. (2017) 174:1290–324. doi: 10.1111/bph.13625
56. Murillo, AG, and Fernandez, ML. Potential of dietary non-Provitamin a carotenoids in the prevention and treatment of diabetic microvascular complications. Adv Nutr. (2016) 7:14–24. doi: 10.3945/an.115.009803
57. Lem, DW, Gierhart, DL, and Davey, PG. A systematic review of carotenoids in the management of diabetic retinopathy. Nutrients. (2021) 13:2441. doi: 10.3390/nu13072441
58. Tan, BL, and Norhaizan, ME. Carotenoids: how effective are they to prevent age-related diseases? Molecules. (2019) 24:1801. doi: 10.3390/molecules24091801
59. Mahmoud, MF, Hassan, NA, El Bassossy, HM, and Fahmy, A. Quercetin protects against diabetes-induced exaggerated vasoconstriction in rats: effect on low grade inflammation. PLoS One. (2013) 8:e63784. doi: 10.1371/journal.pone.0063784
60. Luo, C, Yang, H, Tang, C, Yao, G, Kong, L, He, H, et al. Kaempferol alleviates insulin resistance via hepatic IKK/NF-kappaB signal in type 2 diabetic rats. Int Immunopharmacol. (2015) 28:744–50. doi: 10.1016/j.intimp.2015.07.018
61. Gupta, SK, Dongare, S, Mathur, R, Mohanty, IR, Srivastava, S, Mathur, S, et al. Genistein ameliorates cardiac inflammation and oxidative stress in streptozotocin-induced diabetic cardiomyopathy in rats. Mol Cell Biochem. (2015) 408:63–72. doi: 10.1007/s11010-015-2483-2
62. Li, L, Luo, W, Qian, Y, Zhu, W, Qian, J, Li, J, et al. Luteolin protects against diabetic cardiomyopathy by inhibiting NF-kappaB-mediated inflammation and activating the Nrf2-mediated antioxidant responses. Phytomedicine. (2019) 59:152774. doi: 10.1016/j.phymed.2018.11.034
63. Othman, AI, El-Sawi, MR, El-Missiry, MA, and Abukhalil, MH. Epigallocatechin-3-gallate protects against diabetic cardiomyopathy through modulating the cardiometabolic risk factors, oxidative stress, inflammation, cell death and fibrosis in streptozotocin-nicotinamide-induced diabetic rats. Biomed Pharmacother. (2017) 94:362–73. doi: 10.1016/j.biopha.2017.07.129
64. Chen, YF, Shibu, MA, Fan, MJ, Chen, MC, Viswanadha, VP, Lin, YL, et al. Purple rice anthocyanin extract protects cardiac function in STZ-induced diabetes rat hearts by inhibiting cardiac hypertrophy and fibrosis. J Nutr Biochem. (2016) 31:98–105. doi: 10.1016/j.jnutbio.2015.12.020
65. Kumar, B, Gupta, SK, Srinivasan, BP, Nag, TC, Srivastava, S, Saxena, R, et al. Hesperetin rescues retinal oxidative stress, neuroinflammation and apoptosis in diabetic rats. Microvasc Res. (2013) 87:65–74. doi: 10.1016/j.mvr.2013.01.002
66. Tsai, SJ, Huang, CS, Mong, MC, Kam, WY, Huang, HY, and Yin, MC. Anti-inflammatory and antifibrotic effects of naringenin in diabetic mice. J Agric Food Chem. (2012) 60:514–21. doi: 10.1021/jf203259h
67. Ahad, A, Ganai, AA, Mujeeb, M, and Siddiqui, WA. Chrysin, an anti-inflammatory molecule, abrogates renal dysfunction in type 2 diabetic rats. Toxicol Appl Pharmacol. (2014) 279:1–7. doi: 10.1016/j.taap.2014.05.007
68. Homayouni, F, Haidari, F, Hedayati, M, Zakerkish, M, and Ahmadi, K. Blood pressure lowering and anti-inflammatory effects of hesperidin in type 2 diabetes; a randomized double-blind controlled clinical trial. Phytother Res. (2018) 32:1073–9. doi: 10.1002/ptr.6046
69. Hadi, S, Alipour, M, Aghamohammadi, V, Shahemi, S, Ghafouri-Taleghani, F, Pourjavidi, N, et al. Improvement in fasting blood sugar, anthropometric measurement and hs-CRP after consumption of epigallocatechin3-gallate (EGCG) in patients with type 2 diabetes mellitus. Nutr Food Sci. (2020) 50:348–59. doi: 10.1108/NFS-04-2019-0126
70. Stote, KS, Wilson, MM, Hallenbeck, D, Thomas, K, Rourke, JM, Sweeney, MI, et al. Effect of blueberry consumption on Cardiometabolic health parameters in men with type 2 diabetes: an 8-week, double-blind, randomized, placebo-controlled trial. Curr Dev Nutr. (2020) 4:nzaa030. doi: 10.1093/cdn/nzaa030
71. Fallahzadeh, MK, Dormanesh, B, Sagheb, MM, Roozbeh, J, Vessal, G, Pakfetrat, M, et al. Effect of addition of silymarin to renin-angiotensin system inhibitors on proteinuria in type 2 diabetic patients with overt nephropathy: a randomized, double-blind, placebo-controlled trial. Am J Kidney Dis. (2012) 60:896–903. doi: 10.1053/j.ajkd.2012.06.005
72. Zakerkish, M, Jenabi, M, Zaeemzadeh, N, Hemmati, AA, and Neisi, N. The effect of Iranian Propolis on glucose metabolism, lipid profile, insulin resistance, renal function and inflammatory biomarkers in patients with type 2 diabetes mellitus: a randomized double-blind clinical trial. Sci Rep. (2019) 9:7289–8. doi: 10.1038/s41598-019-43838-8
73. Forte, R, Cennamo, G, Bonavolonta, P, Pascotto, A, de Crecchio, G, and Cennamo, G. Long-term follow-up of oral administration of flavonoids, Centella asiatica and Melilotus, for diabetic cystoid macular edema without macular thickening. J Ocul Pharmacol Ther. (2013) 29:733–7. doi: 10.1089/jop.2013.0010
74. Takano, K, Nakaima, K, Nitta, M, Shibata, F, and Nakagawa, H. Inhibitory effect of ()-epigallocatechin 3-gallate, a polyphenol of green tea, on neutrophil chemotaxis in vitro and in vivo. J Agric Food Chem. (2004) 52:4571–6. doi: 10.1021/jf0355194
75. Mochizuki, K, Tan, Y, Uchiyama, Y, Suzuki, T, Hariya, N, and Goda, T. Supplementation with lower doses of EGCG reduces liver injury markers of type 2 diabetic rats. FundamToxicolSci. (2019) 6:15–23. doi: 10.2131/fts.6.15
76. Sahin, K, and Smith, MO. Regulation of transcription factors by the epigallocatechin-3-gallate in poultry reared under heat stress. Worlds Poult Sci J. (2016) 72:299–306. doi: 10.1017/S0043933916000209
77. Evert, AB, Dennison, M, Gardner, CD, Garvey, WT, Lau, KHK, MacLeod, J, et al. Nutrition therapy for adults with diabetes or prediabetes: a consensus report. Diabetes Care. (2019) 42:731–54. doi: 10.2337/dci19-0014
78. Diabetes prevention program (DPP) research group. The diabetes prevention program (DPP): description of lifestyle intervention. Diabetes Care. (2002) 25:2165–71. doi: 10.2337/diacare.25.12.2165
79. Redan, BW, Buhman, KK, Novotny, JA, and Ferruzzi, MG. Altered transport and metabolism of phenolic compounds in obesity and diabetes: implications for functional food development and assessment. Adv Nutr. (2016) 7:1090–104. doi: 10.3945/an.116.013029
80. Sforcin, JM. Biological properties and Terapeutic applications of Propolis. Phytother Res. (2016) 30:894–905. doi: 10.1002/ptr.5605
81. Tang, L, Zhang, Y, Jiang, Y, Willard, L, Ortiz, E, Wark, L, et al. Dietary wolfberry ameliorates retinal structure abnormalities in db/db mice at the early stage of diabetes. Exp Biol Med. (2011) 236:1051–63. doi: 10.1258/ebm.2011.010400
82. Muriach, M, Bosch-Morell, F, Alexander, G, Blomhoff, R, Barcia, J, Arnal, E, et al. Lutein effect on retina and hippocampus of diabetic mice. Free Radic Biol Med. (2006) 41:979–84. doi: 10.1016/j.freeradbiomed.2006.06.023
83. Kowluru, RA, Menon, B, and Gierhart, DL. Beneficial effect of zeaxanthin on retinal metabolic abnormalities in diabetic rats. Invest Ophthalmol Vis Sci. (2008) 49:1645–51. doi: 10.1167/iovs.07-0764
84. Li, W, Wang, G, Lu, X, Jiang, Y, Xu, L, and Zhao, X. Lycopene ameliorates renal function in rats with streptozotocin-induced diabetes. Int J Clin Exp Pathol. (2014) 7:5008–15.
85. Zheng, Z, Yin, Y, Lu, R, and Jiang, Z. Lycopene ameliorated oxidative stress and inflammation in type 2 diabetic rats. J Food Sci. (2019) 84:1194–200. doi: 10.1111/1750-3841.14505
86. Naito, Y, Uchiyama, K, Aoi, W, Hasegawa, G, Nakamura, N, Yoshida, N, et al. Prevention of diabetic nephropathy by treatment with astaxanthin in diabetic db/db mice. Biofactors. (2004) 20:49–59. doi: 10.1002/biof.5520200105
87. Dong, LY, Jin, J, Lu, G, and Kang, XL. Astaxanthin attenuates the apoptosis of retinal ganglion cells in db/db mice by inhibition of oxidative stress. Mar Drugs. (2013) 11:960–74. doi: 10.3390/md11030960
88. Yeh, PT, Huang, HW, Yang, CM, Yang, WS, and Yang, CH. Astaxanthin inhibits expression of retinal oxidative stress and inflammatory mediators in Streptozotocin-induced diabetic rats. PLoS One. (2016) 11:e0146438. doi: 10.1371/journal.pone.0146438
89. Aydin, EP, and Uyar, ET. Chapter 25-Thioredoxin as an antioxidant protein as a marker in depression In: CR Martin, L Hunter, VB Patel, VR Preedy, and R Rajendram, editors. The Neuroscience of Depression : Academic Press (2021). 251–60.
90. Candas, D, and Li, JJ. MnSOD in oxidative stress response-potential regulation via mitochondrial protein influx. Antioxid Redox Signal. (2014) 20:1599–617. doi: 10.1089/ars.2013.5305
91. Kops, GJ, Dansen, TB, Polderman, PE, Saarloos, I, Wirtz, KW, Coffer, PJ, et al. Forkhead transcription factor FOXO3a protects quiescent cells from oxidative stress. Nature. (2002) 419:316–21. doi: 10.1038/nature01036
92. Li, XN, Song, J, Zhang, L, LeMaire, SA, Hou, X, Zhang, C, et al. Activation of the AMPK-FOXO3 pathway reduces fatty acid-induced increase in intracellular reactive oxygen species by upregulating thioredoxin. Diabetes. (2009) 58:2246–57. doi: 10.2337/db08-1512
93. Görlach, A, Klappa, P, and Kietzmann, T. The endoplasmic reticulum: folding, calcium homeostasis, signaling, and redox control. Antioxid Redox Signal. (2006) 8:1391–418. doi: 10.1089/ars.2006.8.1391
94. Rutter, GA, and Leclerc, I. The AMP-regulated kinase family: enigmatic targets for diabetes therapy. Mol Cell Endocrinol. (2009) 297:41–9. doi: 10.1016/j.mce.2008.05.020
95. Biessels, GJ, van der Heide, LP, Kamal, A, Bleys, RL, and Gispen, WH. Ageing and diabetes: implications for brain function. Eur J Pharmacol. (2002) 441:1–14. doi: 10.1016/s0014-2999(02)01486-3
96. Li, ZG, Zhang, W, Grunberger, G, and Sima, AA. Hippocampal neuronal apoptosis in type 1 diabetes. Brain Res. (2002) 946:221–31. doi: 10.1016/s0006-8993(02)02887-1
97. Hu, BJ, Hu, YN, Lin, S, Ma, WJ, and Li, XR. Application of lutein and Zeaxanthin in nonproliferative diabetic retinopathy. Int J Ophthalmol. (2011) 4:303–6. doi: 10.3980/j.issn.2222-3959.2011.03.19
98. Moschos, MM, Dettoraki, M, Tsatsos, M, Kitsos, G, and Kalogeropoulos, C. Effect of carotenoids dietary supplementation on macular function in diabetic patients. Eye Vis (Lond). (2017) 4:23–4. eCollection 2017. doi: 10.1186/s40662-017-0088-4
99. Chous, AP, Richer, SP, Gerson, JD, and Kowluru, RA. The diabetes visual function supplement study (DiVFuSS). Br J Ophthalmol. (2016) 100:227–34. doi: 10.1136/bjophthalmol-2014-306534
100. Zhang, PC, Wu, CR, Wang, ZL, Wang, LY, Han, Y, Sun, SL, et al. Effect of lutein supplementation on visual function in nonproliferative diabetic retinopathy. Asia Pac J Clin Nutr. (2017) 26:406–11. doi: 10.6133/apjcn.032016.13
101. Neyestani, TR, Shariat-Zadeh, N, Gharavi, A, Kalayi, A, and Khalaji, N. The opposite associations of lycopene and body fat mass with humoral immunity in type 2 diabetes mellitus: a possible role in atherogenesis. Iran J Allergy Asthma Immunol. (2007) 6:79–87.
102. Upritchard, JE, Sutherland, WH, and Mann, JI. Effect of supplementation with tomato juice, vitamin E, and vitamin C on LDL oxidation and products of inflammatory activity in type 2 diabetes. Diabetes Care. (2000) 23:733–8. doi: 10.2337/diacare.23.6.733
103. Asemi, Z, Alizadeh, SA, Ahmad, K, Goli, M, and Esmaillzadeh, A. Effects of beta-carotene fortified synbiotic food on metabolic control of patients with type 2 diabetes mellitus: a double-blind randomized cross-over controlled clinical trial. Clin Nutr. (2016) 35:819–25. doi: 10.1016/j.clnu.2015.07.009
104. Coyne, T, Ibiebele, TI, Baade, PD, Dobson, A, McClintock, C, Dunn, S, et al. Diabetes mellitus and serum carotenoids: findings of a population-based study in Queensland. Australia Am J Clin Nutr. (2005) 82:685–93. doi: 10.1093/ajcn.82.3.685
105. Ekesa, B, Nabuuma, D, Blomme, G, and Van den Bergh, I. Provitamin a carotenoid content of unripe and ripe banana cultivars for potential adoption in eastern Africa. J Food Compos Anal. (2015) 43:1–6. doi: 10.1016/j.jfca.2015.04.003
106. Costa, ES, Franca, CN, Fonseca, FAH, Kato, JT, Bianco, HT, Freitas, TT, et al. Beneficial effects of green banana biomass consumption in patients with pre-diabetes and type 2 diabetes: a randomised controlled trial. Br J Nutr. (2019) 121:1365–75. doi: 10.1017/S0007114519000576
107. Guo, XF, Ruan, Y, Li, ZH, and Li, D. Flavonoid subclasses and type 2 diabetes mellitus risk: a meta-analysis of prospective cohort studies. Crit Rev Food Sci Nutr. (2019) 59:2850–62. doi: 10.1080/10408398.2018.1476964
108. Bondonno, NP, Dalgaard, F, Murray, K, Davey, RJ, Bondonno, CP, Cassidy, A, et al. Higher habitual flavonoid intakes are associated with a lower incidence of diabetes. J Nutr. (2021) 151:3533–42. doi: 10.1093/jn/nxab269
109. Adu, MD, Bondonno, CP, Parmenter, BH, Sim, M, Davey, RJ, Murray, K, et al. Association between non-tea flavonoid intake and risk of type 2 diabetes: the Australian diabetes, obesity and lifestyle study. Food Funct. (2022) 13:4459–68. doi: 10.1039/d1fo04209b
110. Chen, M, Wang, K, Zhang, Y, Zhang, M, Ma, Y, Sun, H, et al. New insights into the biological activities of Chrysanthemum morifolium: natural flavonoids alleviate diabetes by targeting α-glucosidase and the PTP-1B signaling pathway. Eur J Med Chem. (2019) 178:108–15. doi: 10.1016/j.ejmech.2019.05.083
111. Zhang, SS, Hou, YF, Liu, SJ, Guo, S, Ho, CT, and Bai, NS. Exploring active ingredients, beneficial effects, and potential mechanism of Allium tenuissimum L. flower for treating T2DM mice based on network pharmacology and gut microbiota. Nutrients. (2022) 14:3980. doi: 10.3390/nu14193980
112. Beydoun, MA, Chen, X, Jha, K, Beydoun, HA, Zonderman, AB, and Canas, JA. Carotenoids, vitamin a, and their association with the metabolic syndrome: a systematic review and meta-analysis. Nutr Rev. (2019) 77:32–45. doi: 10.1093/nutrit/nuy044
113. Sedeek, M, Montezano, AC, Hebert, RL, Gray, SP, Marco, ED, Jha, JC, et al. Oxidative stress, Nox isoforms and complications of diabetes—potential targets for novel therapies. J Cardiovasc Transl Res. (2012) 5:509–18. doi: 10.1007/s12265-012-9387-2
114. Xiao, J. Recent advances in dietary flavonoids for management of type 2 diabetes. Curr Opin Food Sci. (2022) 44:100806. doi: 10.1016/j.cofs.2022.01.002
115. Mihaylova, MM, and Shaw, RJ. The AMPK signalling pathway coordinates cell growth, autophagy and metabolism. Nat Cell Biol. (2011) 13:1016–23. doi: 10.1038/ncb2329
Keywords: flavonoids, carotenoids, diabetic complications, inflammation, oxidative stress
Citation: Jin Y and Arroo R (2023) The protective effects of flavonoids and carotenoids against diabetic complications—A review of in vivo evidence. Front. Nutr. 10:1020950. doi: 10.3389/fnut.2023.1020950
Edited by:
Afolabi Clement Akinmoladun, Federal University of Technology, NigeriaReviewed by:
Dale Brunelle, Grand Forks Human Nutrition Research Center (USDA), United StatesSeong Lin Teoh, National University of Malaysia, Malaysia
Copyright © 2023 Jin and Arroo. This is an open-access article distributed under the terms of the Creative Commons Attribution License (CC BY). The use, distribution or reproduction in other forums is permitted, provided the original author(s) and the copyright owner(s) are credited and that the original publication in this journal is cited, in accordance with accepted academic practice. No use, distribution or reproduction is permitted which does not comply with these terms.
*Correspondence: Yannan Jin, jessica.jin@dmu.ac.uk
†These authors have contributed equally to this work and share first authorship