Calcium Ions Aggravate Alzheimer’s Disease Through the Aberrant Activation of Neuronal Networks, Leading to Synaptic and Cognitive Deficits
- College of Life and Health Sciences, Northeastern University, Shenyang, China
Alzheimer’s disease (AD) is a neurodegenerative disease that is characterized by the production and deposition of β-amyloid protein (Aβ) and hyperphosphorylated tau, leading to the formation of β-amyloid plaques (APs) and neurofibrillary tangles (NFTs). Although calcium ions (Ca2+) promote the formation of APs and NFTs, no systematic review of the mechanisms by which Ca2+ affects the development and progression of AD has been published. Therefore, the current review aimed to fill the gaps between elevated Ca2+ levels and the pathogenesis of AD. Specifically, we mainly focus on the molecular mechanisms by which Ca2+ affects the neuronal networks of neuroinflammation, neuronal injury, neurogenesis, neurotoxicity, neuroprotection, and autophagy. Furthermore, the roles of Ca2+ transporters located in the cell membrane, endoplasmic reticulum (ER), mitochondria and lysosome in mediating the effects of Ca2+ on activating neuronal networks that ultimately contribute to the development and progression of AD are discussed. Finally, the drug candidates derived from herbs used as food or seasoning in Chinese daily life are summarized to provide a theoretical basis for improving the clinical treatment of AD.
Introduction
Alzheimer’s disease (AD) is a neurodegenerative disease with cognitive deficit as the main characteristic (Elgh et al., 2006). During the course of AD development and progression, calcium ion (Ca2+) concentrations are obviously increased in the brains of patients with AD and APP/PS1 Tg mice (Cao et al., 2019). One report has shown that β-amyloid protein (Aβ)1–40 has the ability to increase Ca2+ influx in rat cortical synaptosomes and cultured cortical neurons (MacManus et al., 2000). Similar to Aβ1–40, Aβ1–42 induce the Ca2+ influx via RyRs in primary cultured hippocampal neurons (Marcantoni et al., 2020). Furthermore, the Aβ25–35 peptide promotes Ca2+ influx by activating L- and T-type Ca2+ channels in rat hippocampal slices (Li et al., 2010). The APP intracellular domain (AICD), a APP cleavage fragment, may act as a transcription factor to activate the Ca2+ signaling system (Cao and Südhof, 2001; Leissring et al., 2002). Because of the self-aggregating characteristics of Aβ, Aβ oligomers can promote Ca2+ influx through N-methyl-D-aspartic acid receptor (NMDAR) channels in a short period of time (Kelly and Ferreira, 2006). More directly, Arispe et al. (2010) found that the aggregates of Aβ1–40 and Aβ1–42 form a cation channel on the surface of an artificial lipid membrane that allows the passage of Ca2+. The pore formation ability of Aβ was confirmed and corroborated by atomic force microscopy (Lin et al., 2001), electron microscopy (Lashuel et al., 2002, 2003), and a theoretical model (Durell et al., 1994; Jang et al., 2008).
Reciprocally, Ca2+ is not a passive contributor to the development and progression of AD. In PS-mutant AD brain tissue, a Ca2+ metabolic disorder was evident before the formation of APs or NFTs (Etcheberrigaray et al., 1998), which indicated that the metabolic disorder caused by Ca2+ located in the cytoplasm might be the cause of AD. Based on this hypothesis, previous studies have shown that Ca2+ influx increases the production and aggregation of Aβ and the phosphorylated tau protein, which affects the learning and memory of patients with AD (Etcheberrigaray et al., 1998; Zempel et al., 2010; Tong et al., 2018). Moreover, Ca2+ imbalance leads to dysregulated metabolism that affects many neurophysiological functions related to AD, including the regulation of neuroinflammation, response to neuronal injury, neuronal regeneration, neurotoxicity and autophagy (Wahlestedt et al., 1993; Liu and Zukin, 2007; Decuypere et al., 2011a; Sama and Norris, 2013; Song et al., 2019). These actions of Ca2+ may finally contribute to neuronal death, which results in cognitive decline during the course of AD development and progression.
Given the multiple functions of Ca2+ in AD, its transporters in the cell membrane, endoplasmic reticulum (ER), mitochondria and lysosomes must be involved in regulating the development and progression of AD. As an antagonist of NMDAR, a Ca2+ transporter on the surface of the nerve cell membrane, memantine significantly inhibits Ca2+ influx and was the first Food and Drug Administration (FDA)-approved drug for the treatment of moderate to severe AD in patients (Bullock, 2006). Regarding the important reservoir of Ca2+ in neurons, the ER has been reported to release Ca2+ to the cytosol, which contributes to the development and progression of AD (Guan et al., 2021). Although direct evidence showing the relationship between Ca2+ transport from mitochondria and lysosomes and the learning ability of patients with AD is unavailable, voltage-dependent anion channel protein 1 (VDAC1) is a hub protein that interacts with phosphorylated tau, Aβ, and γ-secretase, and it contributes to their toxic effects on triggering cell death and potentially leading to the dementia that is a characteristic of AD (Shoshan-Barmatz et al., 2018). All this evidence prompted us to summarize the roles of Ca2+ transporters located in different organelles in regulating the development and progression of AD.
Therefore, this review mainly summarizes the molecular mechanisms by which a Ca2+ imbalance in individuals with AD affects the regulation of neuroinflammation, neuronal injury, neuronal regeneration, neurotoxicity, neuroprotection, and autophagy, specifically from the perspective of Ca2+ transporters in the cell, mitochondria, endoplasmic reticulum and lysosomal membranes. By addressing these mechanisms, we will fill the gaps between increased Ca2+ concentrations and the fate of neurons, which results in dementia.
Crosstalk Between Factors Responsible for Ca2+ Dyshomeostasis and Neuroinflammation
Ca2+ Increases the Production of Proinflammatory Cytokines
Neuroinflammation is widely accepted to be mediated by Ca2+ dyshomeostasis and induces the cognitive decline associated with AD. This process is studied to understand the inherent mechanisms by which Ca2+ exerts an effect. For example, Ca2+ increases the production of interleukin (IL)-1β and tumor necrosis factor α (TNF-α) via calcineurin (CaN) in glial cells (Sama and Norris, 2013). Consistently, an indirect blockade of Ca2+ entry into lipopolysaccharide (LPS)-activated microglia stimulates the production of proinflammatory cytokines, such as TNF-α and IL-6 (Dolga et al., 2012). These observations revealed critical roles for Ca2+ in inducing neuroinflammation by concurrently increasing the production of proinflammatory cytokines and decreasing the levels of anti-inflammatory cytokines.
Transporters on the Cell Membrane Mediate the Effects of Ca2+ on the Secretion of Proinflammatory Cytokines
Based on these observations, Ca2+ transporters were found to be involved in regulating neuroinflammation. More specifically, NMDAR is critical for mediating the effects of Ca2+ on stimulating the production of proinflammatory cytokines, such as IL-1β and TNF-α, in primary mouse hippocampal neurons and lamina II neurons of isolated spinal cord slices (Kawasaki et al., 2008; Huang et al., 2011). By deactivating NMDAR, sevoflurane, an NMDAR antagonist, inhibits the production of IL-1β, TNF-α, IL-6, and IL-8, whereas the addition of the NMDAR agonist D-cycloserine restores the suppression of ageing phenotype acquisition in rats (Yang Z. Y. et al., 2020). NMDAR overexpression in primary cultured microglial cells was induced to synthesize nitric oxide (NO) by activating the NF-κB signaling pathway and to exclude the nonspecific action of these pharmacological interventions (Murugan et al., 2011). In the context of inflammation, NMDAR blockade attenuates the clinical symptoms of glutamate excitotoxicity, suggesting that NMDAR exerts potential neuroprotective effects (Wallström et al., 1996). Similar to this observation, blocking the AMPA/kainate receptor also results in the neuroprotection of encephalomyelitis-sensitized mice (Pitt et al., 2000; Smith et al., 2000). Based on this observation, researchers have readily deduced that α-amino-3-hydroxy-5-methyl-4-isoxazole propionate receptor (AMPAR) might also be involved in regulating neuroinflammation. In SG neurons and lamina II neurons isolated from spinal cord slices, AMPAR was reported to mediate Ca2+-stimulated secretion of proinflammatory cytokines, such as IL-1β and TNF-α (Liu et al., 2013). Perampanel, an AMPAR antagonist, concurrently suppressed the expression of proinflammatory cytokines, including IL-1β and TNF-α, and upregulates the expression of anti-inflammatory cytokines, including IL-10 and Transforming Growth Factor Beta 1 (TGF β1), in a rat model of traumatic brain injury (TBI; Chen T. et al., 2017).
In addition to glutamate receptors serving as transporters of Ca2+, some Ca2+ transporters in the cell membrane are reported to be involved in regulating neuroinflammation. For example, the blockade of L-type voltage-gated calcium channels (L-VGCC) by bepridil, nitrendipine or nimodipine attenuates neuroinflammation by deactivating astrocytes and microglial cells in LPS-stimulated or artificial cerebrospinal fluid (aCSF)-injected (i.c.v.) rats and astrocytes from the CA1 region of the hippocampus (Brand-Schieber and Werner, 2004; Daschil et al., 2013; Espinosa-Parrilla et al., 2015; Hopp et al., 2015). These observations were corroborated by the ability of Ca2+ to induce TNF-α production in cultured rat hippocampal neurons through an L-VGCC-dependent mechanism (Furukawa and Mattson, 1998). In addition, transient receptor potential channels (TRPs) have been identified in mammals and are grouped into six families associated with the onset of neurodegenerative diseases of the central nervous system (CNS): vanilloid TRP (TRPV), melastatin TRP (TRPM), ankyrin TRP (TRPA), polycystin TRP (TRPP), and canonical or classical TRP (TRPC) channels (Morelli et al., 2013). Among these channels, TRPM2 deletion suppresses cytokine production by deactivating microglial cells in TRPM2-knockout mice (Miyanohara et al., 2018; Kakae et al., 2019). Activation of the TRPV1 channel increases the production of proinflammatory cytokines, such as IL-6, in microglial cells (Sappington and Calkins, 2008). The roles of TRPV4 in inflammation are still being debated. By blocking TRPV4 channels, the release of IL-1β and TNF-α is inhibited because of the reduced Ca2+ influx, leading to the attenuation of glial cell-mediated inflammation (Shi et al., 2013). In contrast, the opening of TRPV4 channels by a selective TRPV4 agonist, 4α-phorbol 12, 13-didecanoate (4α-PDD), prevents microglial activation and TNF-α release after LPS treatment, and TRPV4 knockdown eliminates the inhibitory effect of agonists on the release of TNF-α from cultured microglial cells (Konno et al., 2012). According to these findings, TRPV4 activation may be induced by microglial cell swelling after activation with LPS. Channel activation may thus serve as an autoregulator to avoid excess microglial activation. In addition, TRPC1-mediated negative regulation may exert an immunosuppressive effect by blocking the initiation of inflammatory pathways in primary microglial cells (Sun Y. et al., 2014; Figure 1). Although Apolipoprotein E4 (APOE4) is not regarded as a canonical Ca2+ transporter, human APOE4 increases the activity of microglial cells by inducing the expression of IL-1β in E4F AD mice (Rodriguez et al., 2014). In contrast to APOE4, other isoforms of APOEs inhibit the synthesis of inflammatory mediators, including COX-2, PGE2, and IL-1β, in primary cultured microglia obtained from the adult rat brain cortex (Chen et al., 2005).
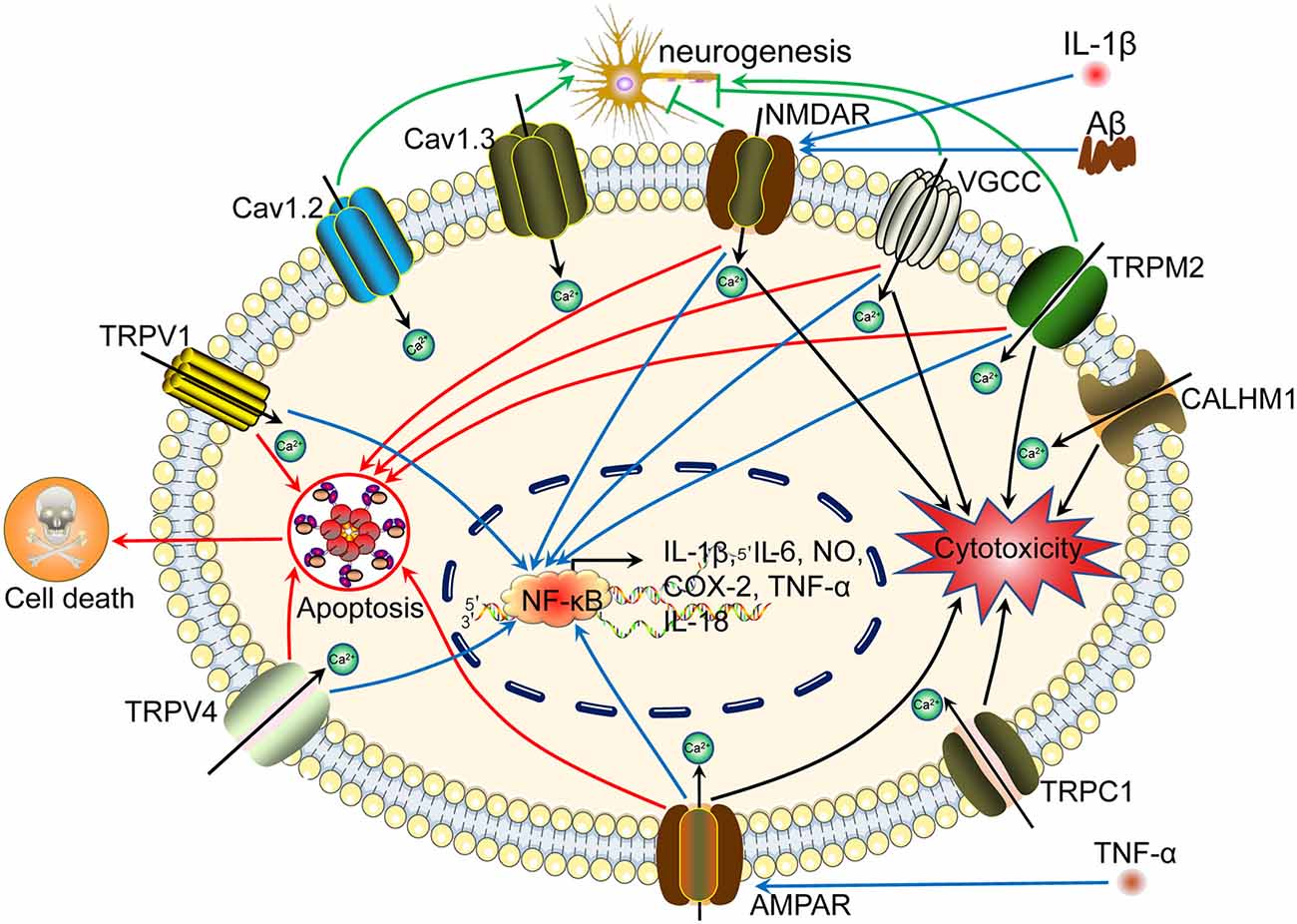
Figure 1. Ca2+ participates in regulating neuroinflammation, neuronal injury, neurogenesis, neurotoxicity, neuroprotection, autophagy and apoptosis via its transporters located on the cell membrane. Aβ activates Ca2+ transporters, including NMDAR, AMPAR, LTCC, Na +/K + -ATPase, CALHM1, TRPV1, and Cav1.2, which promote Ca2+ entry into the cytoplasm and increase the concentration of Ca2+ in neuronal cells. More importantly, these Ca2+ transporters mediate the effects of Ca2+ on neuroinflammation, neuronal injury, neurogenesis, neurotoxicity, neuroprotection, autophagy, and apoptosis through different mechanisms. Aβ activates NMDAR, LTCC, CALHM1, and TRPV1, which result in apoptosis induction, leading to cell death. Regarding neuroinflammation, NMDARs mediate the effects of Aβ on activating NF-κB through a Ca2+-dependent mechanism, which results in transcriptional regulation of the secretion of IL-1β, IL-6, NO, and TNF-α. Moreover, NMDARs induce LC3 II production, leading to autophagy.
The Endoplasmic Reticulum Is Involved in Regulating the Production of Proinflammatory Cytokines and Represents Intracellular Ca2+ Stores
Regarding intracellular stores, genetic ablation of type 2 inositol 1,4,5-triphosphate receptor (InsP3R2) increases the production of cytokines in SOD1G93A mice (Staats et al., 2016). By blocking the activity of Ryanodine Receptor (RyR) with dantrolene, the secretion of inflammatory markers is attenuated because of the deactivation of microglia in LPS-infused rats (Hopp et al., 2015). Treatment with PK11195, a mitochondrial ligand, inhibits store-operated calcium entry (SOCE)-mediated Ca2+ influx, resulting in the downregulation of COX-2 expression in human microglial cells (Hong et al., 2006). Thus, the endoplasmic reticulum (ER), as an intracellular Ca2+ store, is critical for regulating neuroinflammation via InsP3R-, RyR- and SOCE-dependent mechanisms. Interferon α/β (IFNα/β) induce cell apoptosis through Ca2+ release-activated Ca2+ (CRAC; Yue et al., 2012). As an important component of the mitochondrial permeability transition pore (mPTP), cyclophilin (CypD) knockdown decreases the secretion of proinflammatory cytokines, including Vascular Cell Adhesion Molecule 1 (VCAM-1), IL-6 and TNF-α, in the arteries of mice (Liu et al., 2019; Figure 3).
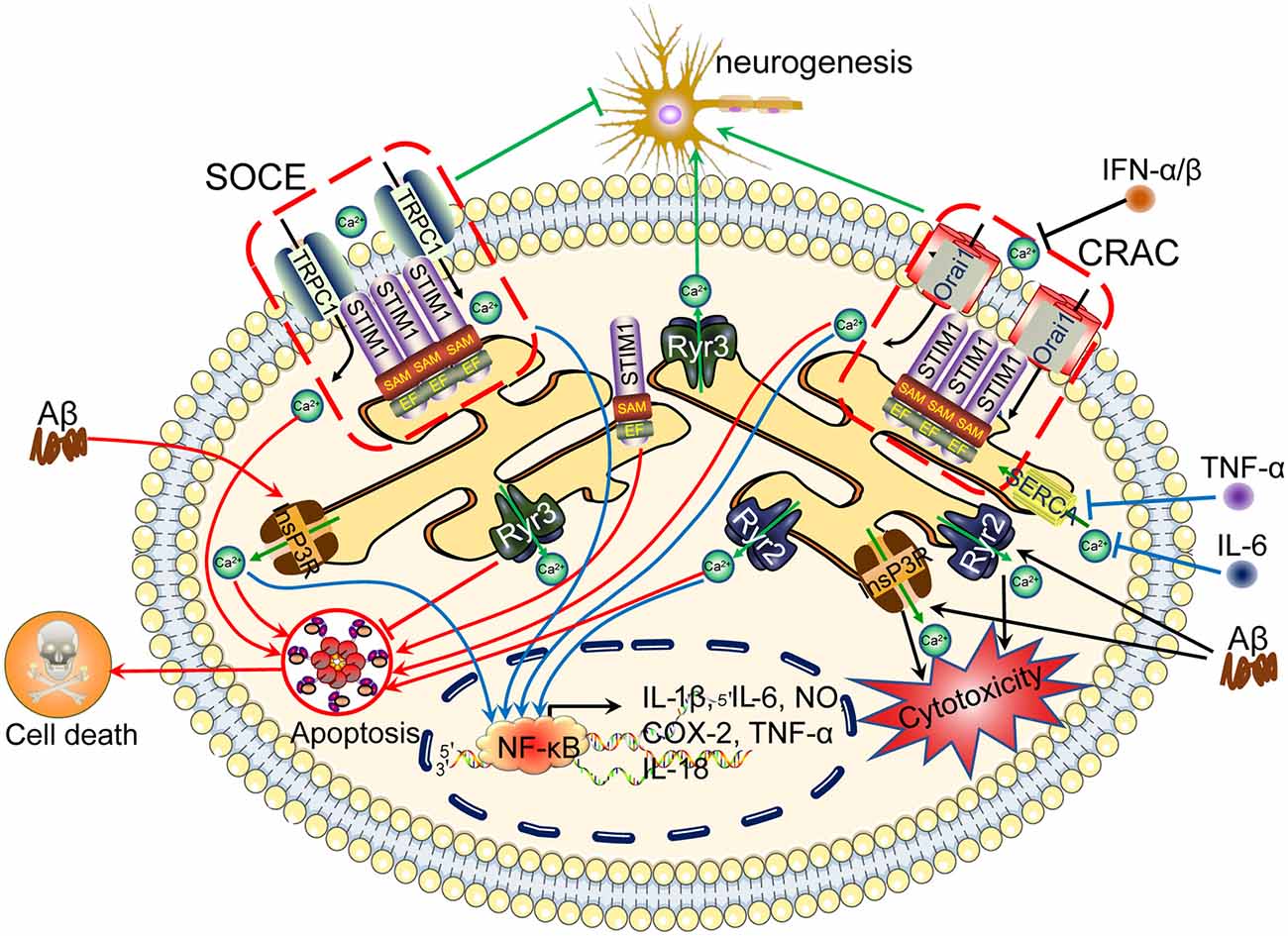
Figure 2. Ca2+ channels in the ER are involved in regulating neuroinflammation, apoptosis, tau phosphorylation and Aβ deposition, leading to cognitive impairment. The accumulation of Aβ in neuronal cells induces Ca2+ influx from the intracellular Ca2+ store, namely, the ER. In addition, Ca2+ depletion from the ER triggers sustained extracellular Ca2+ influx to the cytosol via a SOCE pathway, including TRPC1 and Orai1, by activating the Stim. During these processes, InsP3R and RyR2 play important roles in inducing Ca2+ influx from the ER to the cytosol, regulating apoptosis, neurogenesis, tau phosphorylation and Aβ deposition and subsequently leading to cognitive impairment. ER, endoplasmic reticulum; SOCE, store-operated calcium entry.
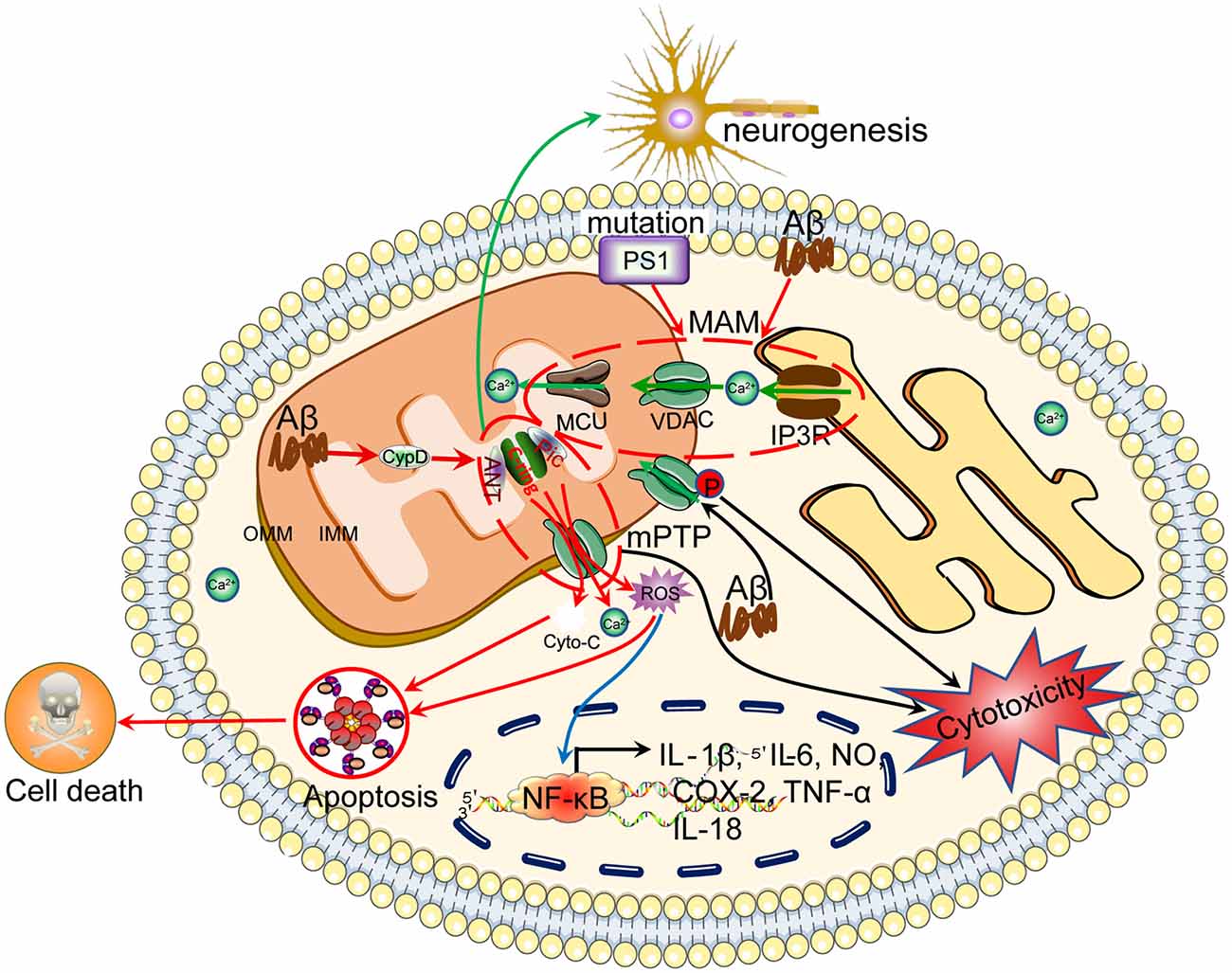
Figure 3. Ca2+ efflux from mitochondria regulates the apoptosis of neuronal cells, which results in cognitive dysfunction. Ca2+ is transported to the mitochondria via MCU. Under physiological or pathological conditions, Ca2+ is continuously shuffled between the ER and mitochondria via VDAC. Moreover, Ca2+ in mitochondria induces the formation of the mPTP, which transports Ca2+ and small molecules, such as ROS and cytochrome C, from the mitochondria to the cytosol, leading to neuronal apoptosis. The loss of neurons will cause cognitive dysfunction.
With opposite effects, proinflammatory cytokines have the ability to modulate the Ca2+ balance via their transporters. For example, TNF-α, IL-1β, and IFNγ increase the influx of Ca2+ into microglial cells, which indicates crosstalk between Ca2+ and neuroinflammatory factors in cultured hippocampal neurons (Goghari et al., 2000; McLarnon et al., 2001; Franciosi et al., 2002). IL-1β increases the expression of AMPAR on the cell surface, which potentially contributes to the entry of Ca2+ into hippocampal neurons (Viviani et al., 2003; Simões et al., 2012). In contrast to AMPAR, IL-1β inhibits L-VGCC activity by suppressing the protein expression of Ca2+ channels in primary cultured neurons (Zhou et al., 2006; Zhou, 2010). In addition, IL-1β is responsible for increasing the expression of TRPM2, leading to the influx of Ca2+ to microglial cells (Fonfria et al., 2006). Similar to IL-1β, IL-6 potentiates Ca2+ entry through NMDARs in hippocampal neurons (Orellana et al., 2005). Although IL-6 is not expressed in neuronal cells, it downregulates the expression of SERCA2, which blocks Ca2+ entry into the ER, thus maintaining high levels of cytosolic Ca2+ in cardiac myocytes (Villegas et al., 2000). Similar to other cytokines, TNF-α increases Ca2+ currents through NMDARs in cultured rat hippocampal neurons (Furukawa and Mattson, 1998). In addition, TNF-α induces the rapid insertion of AMPAR into the membranes of hippocampal pyramidal neurons (Ogoshi et al., 2005). In addition, the colocalization of GluA1, GluA2 and GluA4 and synaptophysin on the neural crest also indicates the transportation of AMPAR to synapses (Wigerblad et al., 2017). In contrast, TNF-α decreases Ca2+ influx by inhibiting the activity of L-VGCCs in cultured rat hippocampal neurons and hippocampal CA1 neurons (Furukawa and Mattson, 1998; Sama et al., 2012). Regarding the regulation of intracellular stores, impaired TNF-α signaling disrupts the effects of InsP3R on mediating Ca2+ release from the ER to the cytosol in 3xTg mice (Park et al., 2010). Moreover, calcineurin (CaN) is activated by the proinflammatory cytokine TNF-α in astrocytes (Fernandez et al., 2007; Sama et al., 2008; Furman et al., 2012). TNF-α activates a more complicated mechanism to regulate Ca2+ currents. In addition to TNF-α itself, the TNF-α receptor mobilizes Ca2+ through an RyR-dependent mechanism in cultured neonatal rat dorsal root ganglion (DRG) neurons (Pollock et al., 2002). In addition to proinflammatory cytokines, most investigations have focused on the roles of anti-inflammatory cytokines on Ca2+ transporters. Based on this information, researchers also found that anti-inflammatory cytokines, such as IL-10, reduced the intracellular Ca2+ levels in microglial cells by decreasing Ca2+ release from the ER through the deactivation of the InsP3R-dependent mechanism in cultured hippocampal neurons (Turovskaya et al., 2012). Therefore, the existence of crosstalk between Ca2+ and neuroinflammation will result in the aggravation of AD (Figure 2).
Proinflammatory Cytokines Reciprocally Regulate the Activities of Transporters Expressed on Lysosomes to Regulate the Basal Ca2+ Levels in Glial Cells
In SH-SY5Y cells, IFNγ also induces Ca2+ influx by activating TRPM2, leading to the apoptosis of cultured neurons (Sama et al., 2012). Furthermore, IFNγ reduces the activity of ATPase Sarcoplasmic/Endoplasmic Reticulum Ca2+ Transporting 2b (SERCA2b) in IL-1β-stimulated OSCC cells (Cardozo et al., 2005; Gkouveris et al., 2018). In addition to these cytokines, inflammatory factors, such as H2O2, increase TRPM2 activity, which might lead to increased basal Ca2+ levels in cultured rat microglial cells (Kraft et al., 2004). Poly ADP-ribose polymerase-1 (PARP-1) induces Ca2+ influx by activating TRPM2 in PARP-2 knockout mice (Kraft et al., 2004). All this evidence revealed crosstalk between Ca2+ and neuroinflammatory factors, which aggravates AD via the actions of different transporters (Table 1).
Ca2+ Signaling Impairs Neuronal Function
The Effects of Ca2+ on Impairing Neuronal Functions
Given the crosstalk between Ca2+ and neuroinflammatory factors, we continued to elucidate the roles of Ca2+ in impairing neuronal functions and its effects on the relationship between neuroinflammation and neuronal apoptosis and death (Table 2). For example, accumulating evidence has revealed that appropriate activation of microglial cells may exert beneficial effects by attenuating neuronal apoptosis, increasing neurogenesis, and promoting functional recovery after cerebral ischaemia (Neumann et al., 2008). In contrast, overactivation of microglial cells may result in the apoptosis or death of neurons (Brown and Neher, 2014). Based on these findings, excessive release of Ca2+ initially protects neuronal cells from death by inducing the expression of Bcl-2 through the activated transcription factor NF-κB (Pahl and Baeuerle, 1996; Mattson and Furukawa, 1997), whereas sustained increases in cytosolic Ca2+ concentrations induced by neuronal depolarization result in Aβ1–42 production and subsequent neuronal death (Pierrot et al., 2004). Moreover, a series of studies reviewed in our previous work described the effects of Ca2+ on cell apoptosis via multiple signaling pathways, and this information is not repeated in the present review (Wang and Wang, 2017).
Proinflammatory Cytokines Induce Neuronal Apoptosis or Death via Ca2+ Transporters Located on the Cell Membranes
However, transporters have not been considered critical for mediating the effects of Ca2+ on the apoptosis or death of neurons. Therefore, we further addressed the roles of different types of Ca2+ transporters in regulating the apoptosis or death of neuronal cells, especially during the course of AD development and progression. Due to its close association with neuroinflammation, neuronal apoptosis in the rat hippocampus is induced by IL-1β through an NMDAR-mediated Ca2+ influx mechanism (Dong et al., 2017). By coculturing glial cells with primary hippocampal neurons, IL-1β secreted from glial cells triggers neuronal death via tyrosine phosphorylation and NMDAR trafficking mechanisms (Viviani et al., 2006; Dong et al., 2017). In contrast to the action of IL-1β, IL-6 reduces Ca2+ overload by deactivating NMDARs, which resulted in the death of cultured cerebellar granule neurons (CGNs) via the JAK/CaN pathways (Ma et al., 2015). As another type of glutamate receptor involved in Ca2+ transport, AMPAR, which is trafficked to the plasma membrane, mediates the effects of TNF-α on exacerbating the effects of spinal cord injury on cell death (Ferguson et al., 2008; Beattie et al., 2010). By inhibiting the activities of L-VGCC, Gas6 or nimodipine suppresses Aβ-induced neuronal apoptosis by attenuating Ca2+ influx into primary cultured cortical and hippocampal neurons (Ueda et al., 1997; Yagami et al., 2002). In addition, NMDARs and L-VGCCs mediate the effects of perfluorohexanesulfonate (PFHxS) on activating the AMPK and ERK pathways, leading to the apoptosis of P12 cells (Lee et al., 2016). Among the Ca2+ transporters located in the cell membrane, TRPV1 overexpression disrupts mitochondrial function and induces cytochrome c release, which results in the death of a human microglial cell line (HMO6; Kim et al., 2006; Zhang and Liao, 2015). Similarly, ectopically expressed TRPV4 in glial cells induces neuronal damage via an apoptotic mechanism (Shi et al., 2013). Consistent with these findings, pharmacological or genetic interventions targeting TRPV4 suppress neuronal cell death by decreasing the expression of proinflammatory cytokines, such as IL-1β and TNF-α (Konno et al., 2012; Shi et al., 2013). As another type of TRP family protein, TRPM2 is activated to induce Ca2+ influx, resulting in the death of RIN-5F rat insulinoma cells and rat cortical neurons (Kaneko et al., 2006). TRPM2 knockdown reduces the toxicity of Aβ and subsequent death of primary rat neuron cultures (Fonfria et al., 2005; Li and Jiang, 2018; Figure 1).
Ca2+ Transporters Located on the ER Membrane Are Responsible for Regulating Neuronal Apoptosis
Although APOE4 is not a canonical Ca2+ transporter, APOE4 overexpression induces Ca2+ influx, resulting in neuronal apoptosis (Veinbergs et al., 2002; Jiang et al., 2015). Through a more complicated mechanism, APOE4 induces neuronal apoptosis in APOE4 knockout mice by activating NMDAR-mediated Calcium/Calmodulin dependent protein kinase II (CaMKII) pathways (Qiao et al., 2017). Moreover, TBI induces apoptosis in the cortex and hippocampus of Tg mice overexpressing human APOE4 by activating APOE4 (Giarratana et al., 2020). In addition, ER stress also mediates the effects of the unfolded protein response (UPR) and misfolded proteins on inducing apoptosis through mechanisms related to Ca2+ influx (Nishitoh et al., 2009; Moreno et al., 2013). Specifically, Ca2+ transporters located on the ER membrane, including InsP3R and RyR, are reported to be involved in regulating neuronal apoptosis. For example, type 3 InsP3R regulates cell death by modulating Ca2+ release from the ER to the cytosol in postnatal cerebellar granule cells (Blackshaw et al., 2000; Wang and Zheng, 2019). Isoflurane treatment induces Ca2+ influx, leading to caspase-3 activation by cleavage in DT40 cells (Joseph et al., 2014). Upon the stimulation of P2X7R by isoflurane and sulforaphane, InsP3R mediates the effects of Ca2+ on inducing apoptosis or cell death of NG108-15 and PC12 neuronal cells and cells in nude mice (Wei et al., 2008; Chao et al., 2012; Hudecova et al., 2016). Specifically, Aβ25–35 induces the apoptosis of murine astrocytes via InsP3R- and Ca2+-activating pathways (Oseki et al., 2014). In addition to InsP3R, the posttranslational modification of RyR2 by S-glutathionylation increases channel activity, resulting in the death of rat cortical neurons (Bull et al., 2008). In contrast, the suppression of RyR3 expression in TgCRND8 neurons increases the neuronal death rate, which suggests a protective role for RyR in the late stages of AD pathogenesis (Supnet et al., 2010).
Based on these observations, ethanol dose-dependently increases the intracellular Ca2+ concentration, which damages HepG2 hepatocytes by upregulating the expression of the Orai1 and Stromal interaction molecule 1 (Stim1) mRNAs and proteins (Liu et al., 2012). Although the pathophysiological effects of decreased Store-operated calcium entry (SOCE) levels in AD remain unclear, several lines of evidence have shown that SOCE deficits lead to neuronal cell death and decreased synaptic plasticity (Soboloff and Berger, 2002; Calvo-Rodriguez et al., 2020). As expected, Stim1 silencing alleviates the apoptosis of H2O2-treated endothelial progenitor cells (Wang et al., 2016). Moreover, the downregulation of Stim1 by an siRNA concurrently increases neuronal viability and inhibits apoptotic cell death by decreasing the intracellular Ca2+ levels (Selvaraj et al., 2016). In PC3 and DU145 cells, both Stim1 and Orai1 separately mediate the effects of resveratrol (RSV), a natural polyphenol, on activating autophagic cell death (Selvaraj et al., 2016). In addition, resveratrol can mediate the release of Ca2+ from intracellular stores (Santoro et al., 2020). As a method to exclude nonspecific effects of pharmacological interventions, silencing the expression of Stim1 and Orai1 reduces the apoptosis rate of LPS-treated pulmonary microvascular endothelial cells by blocking SOCE in pulmonary microvascular endothelial cells (Wang et al., 2016). Researchers excluded the effects of Stim1 on cell apoptosis by transfecting Orai1 mutants and observed decreases in both SOCE and the rate of thapsigargin-induced apoptosis in human prostate cancer (PCa) cells (Flourakis et al., 2010; Figure 2).
Mitochondrial Dysfunction Is Also Involved in Mediating the Effects of Ca2+ on Neuronal Apoptosis
However, ER stress is not the only mechanism by which the effects of Ca2+ on neuronal apoptosis are mediated: mitochondrial dysfunction is also reported to be involved in this process (Yoon et al., 2011). Consistently, Stim1 or Orai1 knockdown attenuates the intracellular Ca2+ overload, restores the mitochondrial membrane potential, decreases the release of cytochrome c and inhibits ethanol-induced apoptosis (Cui et al., 2015). Without affecting ER stress, curcumin protects mitochondria from oxidative damage by attenuating the apoptosis of cortical neurons (Zhu et al., 2004). In primary cultured spinal neurons, salidroside (Sal) treatment decreases apoptosis by activating PINK-Parkin pathways, leading to mitophagy of mitochondria (Gu et al., 2020). Similar to its effects on AD, Aβ1–42 induces neuronal apoptosis by concurrently upregulating mitochondrial fission protein dynamin-related protein 1 (Drp1) and downregulating mitofusin 1/2 (Mfn1/2) and dynamin-like GTPase (OPA-1) in primary cultures of mouse cerebral cortical neurons (Han et al., 2017). In addition, Aβ25–35 induces cytochrome c-mediated apoptosis of NT2 cells through a functional mitochondria-dependent mechanism (Morais Cardoso et al., 2002). In this mechanism, Ca2+ transport by InsP3R to mitochondria induced by opening the mPTP induces the release of cytochrome c, which results in the apoptosis of cells (Szalai et al., 1999). In fact, mPTP opening induces matrix swelling, the subsequent rupture of the outer membrane, and nonspecific release of proteins in the intermembrane space into the cytosol upon cannabidiol (CBD) induction of human monocyte apoptosis (Wu et al., 2018). By inhibiting the opening of the mPTP in mitochondria, mortalin overexpression blocks Aβ-induced SH-SY5Y cell apoptosis (Qu et al., 2012). In AD mice, CyPD knockout decreases the cell death rate by attenuating the opening of the mPTP in mitochondria (Du et al., 2008; Pahrudin Arrozi et al., 2020).
VDAC1 expression induces cell death and apoptosis by activating the Ca2+ signaling cascade in A549 cells (Weisthal et al., 2014). VDAC is involved in the apoptosis of lymphoblastoid cells carrying a mitochondrial DNA mutation (Yuqi et al., 2009). Through a direct interaction with Bax, VDAC induces the transport of cytochrome c through membranes (Shimizu et al., 1999). Moreover, the cleavage of the pro-apoptotic protein Bid by caspase-8 induces the closure of VDAC, which leads to protein release from mitochondria and apoptosis (Rostovtseva et al., 2004). In contrast, Bcl-xL promotes the opening of the VDAC, which results in a reduced apoptosis rate of cultured FL5.12 cells (Vander Heiden et al., 2001; Bessou et al., 2020). Fatty acid binding protein 5 (FABP5), which is expressed in oligodendrocytes, induces mitochondrial macropore formation through VDAC-1 and Bax, thus accelerating mitochondria-induced glial cell death. These two proteins mediate mitochondrial outer membrane permeability, resulting in the release of mitochondrial DNA and cytochrome c into the cytoplasm and activation of apoptotic caspases (Cheng et al., 2020). More interestingly, BAPTA-AM, a Ca2+-chelating reagent, inhibits mitochondria-mediated apoptosis by decreasing the oligomerization of VDAC1 in HeLa and T-REx-293 cells (Keinan et al., 2013). Consistent with this observation, anion transport inhibitors, including 4’-diisothiocyano-2,2’-stilbenedisulfonic acid (DIDS), SITS, H2DIDS, DNDS, and DPC, inhibit apoptosis-associated VDAC1 oligomerization (Ben-Hail and Shoshan-Barmatz, 2016). In addition, blockade of plasmalemmal VDAC1 with a specific antibody suppresses Aβ-induced neuronal apoptosis (Thinnes, 2011; Lim et al., 2021a). In THP-1 macrophages, DIDS disodium salt, an inhibitor of VDAC1, attenuates the apoptosis of THP-1 macrophages by decreasing intracellular Ca2+ levels (Chen et al., 2014). Similarly, Ca2+ transporters generally mediate the regulatory effects of Ca2+ on neuronal apoptosis, especially in the context of AD (Figure 3).
Ca2+ Inhibits The Regulation of Neuronal Stem Cells
Ca2+ Modulates Neuronal Differentiation, Migration and Self-renewal During the Course of Neurogenesis
During the course of AD development and progression, neurogenesis is markedly inhibited in the brains of patients with AD and mouse models (Rash et al., 2016). Given the potential roles of Ca2+ in AD, we summarize the effects of Ca2+ on neurogenesis during the course of AD development and progression. Indeed, higher frequencies of Ca2+ oscillations increase the differentiation of hippocampus-derived neural stem cells (NSCs) into neurons in adult rats (Wang Q. et al., 2015). Moreover, Epac2 mediates PACAP-induced differentiation of neural progenitor cells (NPCs) into astrocytes along with an increase in intracellular Ca2+ levels, which also activated the signaling pathway for astrocytogenesis in Epac2-knockout (KO) mice (Seo and Lee, 2016). NSC differentiation is closely related to the expression of VGCC, especially Caveolin 1 (Cav1) through regulating Ca2+ influx (D’Ascenzo et al., 2006). Moreover, exposure in extremely low-frequency electromagnetic fields (ELFEF) promotes the differentiation of NSCs by upregulating the expression and function of Cav1 (Piacentini et al., 2008c). Furthermore, bidirectional radial Ca2+ activity elongates the fiber of radial glial cells (RGCs) and simultaneously induces neurogenesis during early cortical column development (Rash et al., 2016). By upregulating the Notch signaling pathway after brain injury, Ca2+ waves generated in neighboring astrocytes propagate to NPCs, inducing neurogenic behavior, including the self-renewal and migration of progenitor cells (Kraft et al., 2017). Based on these observations, Ca2+ induces neuronal differentiation, migration and self-renewal during the course of neurogenesis.
Ca2+ Transporters Located on the Cell Membranes Are Required for Neurogenesis
Given the key roles of Ca2+ in neurogenesis, its transporters are also required for neurogenesis. In the developing cerebellum, granule cell precursors differentiate upon activation of a homodimeric G protein-coupled receptor that is sensitive to Ca2+ levels called calcium-sensing receptor (CaSR). CaSR activation in vivo induces the homing of granule cell precursors during differentiation, mainly through CaSR interactions with integrin complexes (Tharmalingam et al., 2016). Among these CaSRs, the lower activity of NMDARs in NR1+/– mice contributes to increased cell proliferation and neurogenesis compared to the activity in the brains of adult NR1+/+ mice (Bursztajn et al., 2007). In contrast, intraperitoneal injection of the NMDAR agonist NMDA (2 mg/kg/day) promotes cell proliferation in the subventricular zone (SVZ) of rats (Fan et al., 2012). Unfortunately, the researchers did not extend their investigations to Ca2+, although NMDAR affects neurogenesis. Compared to NMDARs, the roles of AMPARs in neurogenesis are relatively simple. In rats administered chronic corticosterone (CORT), S47445, a novel AMPAR-positive allosteric modulator (AMPA-PAM), exerted significant neurogenic effects on the proliferation, survival and maturation of new hippocampal neurons (Mendez-David et al., 2017). Moreover, AMPAR mediates kainate-induced radial glia-like stem cell proliferation (Shtaya et al., 2018). Human NPCs contain Ca2+-permeable AMPARs; however, AMPARs were engineered to become Ca2+-impermeable receptors during the course of differentiation from NPCs to neurons or astrocytes through RNA editing of the AMPA receptor subunit GluR2 at the Q/R site (Whitney et al., 2008). Then, the NMDAR subunits NR1 and NR2B and the AMPAR subunit GluR2 in Ca2+-impermeable AMPARs were upregulated at the mRNA level in differentiated neuroepithelial precursors, indicating their likely contribution to neurotransmission after first establishing neuronal networks (Muth-Köhne et al., 2010; Wang et al., 2018).
In addition to NMDARs and AMPARs, different types of VGCCs and TRPs in cell membranes are also involved in regulating neurogenesis. For example, the differentiation of dental pulp stem cells (DPSCs) into neural cells is markedly inhibited by regulating the levels of the distal C-terminus (DCT) upon treatment with nimodipine and knock down of Cav1.2 expression (Ju et al., 2015). In the dentate gyrus (DG) region, deletion of Cav1.2 decreases the numbers of doublecortin-positive adult-born neurons, suggesting important roles for Cav1.2 in adult neurogenesis (Temme et al., 2016). Consistent with these findings, Cav1.3 knockout impairs hippocampal neurogenesis and inhibits neuronal differentiation (Marschallinger et al., 2015). More importantly, Ca2+ mediates the effects of L-VGCC on the neurogenesis of interneurons in nifedipine-treated NPCs (Brustein et al., 2013). Similar to L-VGCCs, blockade of other types of VGCCs, such as N- and T-VGCCs, decreases the migration and neurite extension of developing neurons (Komuro and Rakic, 1992; Louhivuori et al., 2013). On the other hand, TRPs are also reported to be involved in regulating neurogenesis. For instance, TRPM2 deficiency results in impaired embryonic neurogenesis because it regulates neural progenitor self-renewal through an SP5-dependent mechanism (Li and Jiao, 2020). In addition, the antisense oligonucleotide-mediated knockdown of TRPC1 expression reduces the effects of bFGF on the proliferation of embryonic rat NSCs (Fiorio Pla et al., 2005; Toth et al., 2016). Blocking SOCE activity with YM-58483 (BPT2) decreases the proliferation of SVZ and neural stem cells (Domenichini et al., 2018). By stereotactically injecting a recombinant adeno-associated virus expressing TRPC1 into the DG of the bilateral hippocampus, we observed that neurogenesis, LTP induction, and cognitive enhancement related to environmental enrichment (EE) were effectively rescued in TRPC1 knockout mice (Du et al., 2017). Consistent with this observation, TRPC3 knockout reduces the effect of Ca2+ on mGluR5-mediated radial glial processes, reducing the neuronal migration rate (Louhivuori et al., 2015; Toth et al., 2016). In addition to these classical Ca2+ transporters in the cell membrane, both APOE1–3 knockout and APOE4 overexpression suppress neurogenic responses in vivo (Hong et al., 2013; Rijpma et al., 2013; Geffin et al., 2017). Based on this evidence, transporters are involved in mediating the effects of Ca2+ on the neurogenesis of NPCs and NSCs (Figure 1).
Intracellular Ca2+ Stores Mediate the Effects of Ca2+ on Neurogenesis
The ER and mitochondria are major intracellular Ca2+ stores and thus mediate the regulatory effects of Ca2+ on neurogenesis. In PC12 cells, ER stress and BDNF-TrkB signaling pathways are involved in the induction of neurogenesis by 3β, 23, 28-trihydroxy-12-oleanene 3β-caffeate from Desmodium sambuense (Cheng et al., 2019). In addition, ER stress mediates the effects of tunicamycin and HRD1 deletion on the aberrant induction of neuronal differentiation and inhibition of dendrite outgrowth in retinoic acid-treated P19 mouse embryonic carcinoma cells (Kawada et al., 2014). More interestingly, transcripts encoding the three main isoforms of the two families of intracellular calcium release channels, namely, InsP3R and RyR, were detected during early neurogenesis in the mouse cerebral cortex (Faure et al., 2001). In particular, an antagonist of the InsP3 pathway, wortmannin, prevents neurogenesis in neural crest cells (Evrard et al., 2004). In addition, Ca2+ waves propagate through radial glial cells in the proliferative cortical ventricular zone (VZ) and require connexin hemichannels, P2Y1 ATP receptors, and intracellular InsP3-mediated Ca2+ release, suggesting critical roles for radial glial signaling mechanisms in cortical neuronal production (Weissman et al., 2004; Lim et al., 2021b). In this process, the G protein-coupled receptor GPR157, an orphan G protein-coupled receptor, is involved in regulating the neuronal differentiation of radial glial progenitors through Gq-InsP3-mediated Ca2+ cascades (Takeo et al., 2016). In mesenchymal stem cells, caffeine, an RyR agonist, induces an intracellular Ca2+ response that increases throughout neuronal differentiation (Resende et al., 2010). Specifically, RyR2 knockout decreases the neurogenesis of embryonic stem cells (Yu et al., 2008). Associated with the aforementioned mechanisms, the proliferation of embryonic and adult NPCs cultured as neurospheres and progenitors in the subventricular zone (SVZ) of adult mice in vivo was attenuated by depleting the expression of Stim1 and Orai1, suggesting pivotal roles for SOCE channel-mediated Ca2+ entry in mammalian neurogenesis (Somasundaram et al., 2014). In addition to Orai1, single knock down of Stim1, a Ca2+ sensor that mediates SOCE, impairs early and late embryonic stem cell differentiation into neural progenitors, neurons or astrocytes, increasing the cell death rate and suppressing the proliferation of neural progenitors (Hao et al., 2014; Deb et al., 2020). Similarly, pharmacological blockade of SOCE decreases the proliferation and self-renewal of NSCs, driving asymmetric division to the detriment of symmetric proliferative division, reducing the population of stem cells in the adult brain, and impairing the ability of SVZ cells to form neurospheres in culture (Domenichini et al., 2018). CRAC channels serve as a major route of Ca2+ entry in NSCs/NPCs and regulate key effector functions, including gene expression and proliferation, indicating that CRAC channels are important regulators of mammalian neurogenesis (Somasundaram et al., 2014). Similar to the ER, mitochondria are intracellular Ca2+ stores involved in regulating the neurogenesis of NPCs. For example, the inhibition of mPTPs and a selective reduction in mitochondrial superoxide spikes significantly ameliorates the negative effects of Aβ1–42 on NPC proliferation and survival (Hou et al., 2014). Moreover, cyclosporin A inhibits neuronal differentiation by suppressing mPTP opening (Hou et al., 2013; Namba et al., 2020). All these observations confirm the involvement of Ca2+ and its transporters in regulating neurogenesis (Table 3).
The Effects of Ca2+ on Neurotoxicity
Ca2+ Induces Excitotoxicity via Its Transporters Located on Cell Membranes
Neurotoxicity might be the inherent cause of the Ca2+-mediated impairment of neuronal functions. In primary cultured cerebral cortical neurons, increased levels of Ca2+ induce excitotoxicity, whereas reduced Ca2+ release exerts neuroprotective effects (Frandsen and Schousboe, 1991). As the natural ligand of NMDAR, NMDA induces neurotoxicity by activating NMDAR in cerebellar granule cells (Xia et al., 1995). In addition to its natural ligand, the exposure of neurons to ethanol and glutamate also induces neurotoxicity by activating NMDARs (Thomas and Morrisett, 2000; Miao et al., 2012). Similar to its effect on the AD pathway, Aβ25–35 induces neurotoxicity by deactivating the pCRMP2 and NMDAR2B signaling pathways in SH-SY5Y cells (Ji et al., 2019). However, the researchers did not extend their observations to the involvement of Ca2+ in neurotoxicity. In cultured cerebellar granule neurons, domoic acid induces neurotoxicity through NMDAR-mediated Ca2+ influx (Berman et al., 2002). By blocking NMDAR-mediated Ca2+ influx, dantrolene and ionomycin prevent neurotoxicity in cultured rat cortical and retinal ganglion cell neurons (Lei et al., 1992). Drug-induced inhibition of Glutamate ionotropic receptor NMDA type subunit 2A (GluN2A) NMDAR or deletion of the GluN2A subunit gene attenuates the effects of homocysteine on increasing intracellular Ca2+ concentrations, leading to neurotoxicity (Deep et al., 2019). In hippocampal neurons, Aβ-induced Ca2+ influx mediated by NMDARs leads to calpain-dependent neurotoxicity (Kelly and Ferreira, 2006; Deep et al., 2019). Based on these observations, NMDARs have the ability to mediate Aβ-induced neurotoxicity via Ca2+-dependent mechanisms. In addition, AMPAR was also reported to be involved in regulating neurotoxicity as another glutamate receptor type functioning as a Ca2+ transporter. For example, cannabinoid receptor activation attenuates the effects of TNF-α on the surface localization of AMPAR, which resulted in excitotoxicity in cultured hippocampal neurons (Zhao et al., 2010; Ganguly et al., 2019). AMPAR trafficking to the cell membrane of CNS neurons regulates excitotoxicity induced by TNF-α (Ferguson et al., 2008). TNF-α induces a rapid reduction in AMPAR-mediated Ca2+ entry by increasing the expression of the GluR2 subunit on the cell surface, which results in excitotoxicity during the progression of neurodegeneration (Rainey-Smith et al., 2010). Moreover, AMPAR mediated AMPA- and kainite-induced neurotoxicity via Ca2+ influx mechanisms in cultured rat hippocampal neurons (Ambrósio et al., 2000). In addition, ethanol induces neurotoxicity in hippocampal slices by activating AMPAR (Gerace et al., 2021). Of note, either Aβ or trimethyltin has the ability to induce neuronal death via activating L-VGCC, leading to the Ca2+ overload (Piacentini et al., 2008a, b). Therefore, NMDARs and AMPARs are critical for inducing neurotoxicity by triggering Ca2+ influx.
In the cell membrane, L-VGCC is also involved in mediating AMPA/Zn2+-induced neurotoxicity in primary cultured rat cortical neurons (Ambrósio et al., 2000; Lee et al., 2016). In these cells, L-VGCCs were further reported to be critical for iron-induced neurotoxicity (Xu Y. Y. et al., 2020). In cerebral cortical cells, CXCL12 induces neurotoxicity via NMDAR and L-VGCC-dependent p38 MAPK activation (Sanchez et al., 2016). By blocking the L/N-type Ca2+ channel, cilnidipine protects the retina from neurotoxicity in ischaemia-reperfusion-treated rats (Sakamoto et al., 2009).
Another family of Ca2+ transporters, TRPs, was also reported to be involved in regulating neurotoxicity. In primary cultures of mouse DRG neurons, the inhibition of TRPV1 with specific blockers, such as capsaicin or resiniferatoxin, reduces the prooxidant capacity of microglial neurotoxicity (Ma et al., 2009). In addition, TRPV1 mediates vanilloid- and low pH-induced neurotoxicity in cultured rat cortical neurons (Shirakawa et al., 2007; Ertilav et al., 2021). In contrast, the inhibition of TRPV1 by the antagonist capsazepine attenuates its neuroprotective effects, indicating that TRPV1 activation contributes to the survival of rat nigral neurons (Park et al., 2012). To the best of our knowledge, no report has reconciled these conflicting results. With respect to TRPC1, neurotoxicity in SH-SY5Y cells is markedly induced by treatment with 1-methyl-4-phenylpyridinium ion (MPP+) through TRPC1-deactivating Ca2+-dependent mechanisms (Bollimuntha et al., 2005). TRPC1 overexpression inhibits neurotoxicity by inhibiting the release of cytochrome c and the expression of the Bax and Apaf-1 proteins in SH-SY5Y cells (Morelli et al., 2013). In contrast to TRPC1, TRPC6 deletion attenuates the effects of NMDAR-mediated Ca2+ entry, resulting in a disruption of the effect of Ca2+ on neurotoxicity in primary cultured neurons (Chen J. et al., 2017). Blocking TRPV4-mediated Ca2+ influx reduces the neurotoxicity of paclitaxel to small and medium dorsal root ganglion neurons (Boehmerle et al., 2018). Regarding TRPM2, cisplatin-induced neurotoxicity in primary DRG cells is attenuated by treatment with its antagonist, 2-aminoethoxydiphenyl borate (Chen J. et al., 2017). TRPM2 knockout blocks Aβ oligomer-induced neurotoxicity, which results in impaired memory in APP/PS1 mice (Ostapchenko et al., 2015). In hippocampal neurons, Aβ1–42 induces neurotoxicity by activating TRPM2 (Li and Jiang, 2018).
In addition to these canonical Ca2+ transporters, decreasing the expression of CALHM1 exerts neuroprotective effects on oxygen and glucose deprivation in hippocampal slices (Garrosa et al., 2020). On the other hand, APOE has been reported to be involved in regulating neurotoxicity. For example, APOE4 promotes the neurotoxicity induced by Aβ aggregation in AD (Ma et al., 1996). Extracellular APOE4 is cytotoxic to human neuroblastoma SK-N-SH cells, and Aβ1–42 enhances the cytotoxicity of APOE4. The carboxyl terminal mutation of L279Q, K282A or Q284A decreases the ability of APOE4 to form SDS-stable oligomers and decreases its cytotoxicity. Structural and thermodynamic analyses showed that all three APOE4 mutants contain significantly increased α-helical and β-sheet structures, which resulted in reduced exposure of the hydrophobic surface to the solvent and reduced conformational stability during chemical denaturation (Dafnis et al., 2018). In N2a-APP695 cells, APOE4 exacerbates the effects of ethanol on inducing neurotoxicity by increasing oxidative stress and apoptosis (Ji et al., 2019). In contrast, APOE1–3 has been shown to protect primary cultures of rat cortical neurons from the neurotoxic effects of the nonfibrillar C-terminal domain of Aβ (Drouet et al., 2001; Brookhouser et al., 2021). APOE isoforms play different roles in neurotoxicity by modulating Aβ deposition in the mouse brain (Drouet et al., 2001). Ca2+ mediates the effects of truncated APOE on neurotoxicity in cultured embryonic rat hippocampal neurons (Tolar et al., 1999). Through these mechanisms, APOE-related neurotoxicity might be a therapeutic target for AD (Marques and Crutcher, 2003; Figure 1).
The ER Mediates the Effects of Ca2+ on Inducing Neurotoxicity as an Intracellular Store
Since Ca2+ regulates neurotoxicity via transporters located in the cell membrane, the roles of Ca2+ derived from intracellular stores in neurotoxicity are further addressed in Table 4. For example, Aβ induces neurotoxicity in cortical neurons via an ER-mediated apoptotic pathway (Ferreiro et al., 2006; Goswami et al., 2020). In the spinal cord, Ca2+ mediates the effects of ER stress on neurotoxicity (Li et al., 2014). By alleviating ER stress, nicotine suppresses the activity of MPP + /MPTP associated with neurotoxicity in PC12 cells (Cai et al., 2017). Similar to its role in AD, Aβ induces neurotoxicity in cortical neurons by promoting ER stress (Song et al., 2008).
As Ca2+ mediates the effects of ER stress on neurotoxicity, Ca2+ transporters in ER membranes must be associated with neurotoxicity. For example, The generation of InsP3 by activated M3 muscarinic receptors contributes to increased Ca2+ influx and subsequent cytotoxicity in rat cerebellar granule cells (Limke et al., 2004). Furthermore, cyanide induces the formation of InsP3, which triggers intracellular neurotoxic signaling events in PC12 cells (Yang et al., 1996). In hippocampal neurons, Ca2+ was also found to be the critical cause of microcystin-LR-induced neurotoxicity through PLC- and InsP3-dependent pathways (Cai et al., 2015). Regarding the receptors of InsP3, InsP3R triggers Ca2+ influx to mediate isoflurane-induced neurotoxicity, which is facilitated by an APP mutant in SH-SY5Y cells (Liu et al., 2016). In primary cultures of cortical cells, Aβ induces neurotoxic effects by inducing Ca2+ release from the ER via InsP3R- and RyR-dependent mechanisms (Ferreiro et al., 2004). After inhibiting the activity of InsP3R and RyR, the cytotoxicity and increased Ca2+ levels are attenuated. More interestingly, the combined inhibition of both receptors paradoxically increases the amount of cytosolic Ca2+ entering PC12 cells from the extracellular space, increasing cytotoxicity (Yang et al., 2019). In addition to InsP3R, RyR alone might be critical for modulating neurotoxicity in human microglia and THP-1 cells (Klegeris et al., 2007; Holland and Pessah, 2021). In cultured mammalian neurons, Xbpls ameliorates Aβ-induced neurotoxicity through an RyR-dependent mechanism (Fernandez-Funez et al., 2010). Thus, the ER is an important intracellular Ca2+ store for regulating neurotoxicity in neurons (Figure 2).
Mitochondria Are Critical for Regulating Neurotoxicity Through a Ca2+-Dependent Mechanism
In addition to the ER, mitochondria are reported to be critical for regulating neurotoxicity through a Ca2+-dependent mechanism. In particular, VDAC1, a transporter located in mitochondria, mediates Aβ-induced neurotoxicity in PC12 and SH-SY5Y cells and thus represents a potential target for AD treatment (Smilansky et al., 2015). In addition, the dephosphorylation of VDAC1 by hesperidin blocks Aβ-induced neurotoxicity in PC12 cells through a mitochondria-dependent mechanism (Wang et al., 2013). Aβ directly induces neurotoxicity via the dephosphorylation of VDAC1 in murine septal SN56, SH-SY5Y and hippocampal HT22 cells (Fernandez-Echevarria et al., 2014; Shoshan-Barmatz et al., 2018). In these cells, the interaction between VDAC and mERα at the plasma membrane may lead to the modulation of Aβ-induced neurotoxicity (Marin et al., 2007). In addition to VDAC1, an anti-VDAC2 antibody reduces neurotoxicity by decreasing intracellular Ca2+ levels in SH-SY5Y cells (Marin et al., 2007; Nagakannan et al., 2019). By inhibiting the opening of the mPTP, cyclosporin A protects SH-SY5Y and PC12 cells from neurotoxicity (Ye et al., 2016). In primary cultures of rat cortical neurons, 4-hydroxy-2(E)-nonenal facilitates NMDA-induced neurotoxicity by opening the mPTP, which results in Ca2+ influx (Choi et al., 2013). This observation is further supported by a report showing that NMDA induced neurotoxicity via the mPTP in cultured murine cortical neurons (Kinjo et al., 2018). Based on this evidence, intracellular Ca2+ stores are involved in mediating the effects of Ca2+ on neurotoxicity, which potentially contributes to neuronal apoptosis or death (Table 4, Figure 3).
Ca2+ Disrupts The Autophagic Clearance of Aggregated Proteins
Ca2+ Transporters on the Cell Membranes Are Presumably Involved in Regulating Autophagy and Are Responsible for Clearing Aβ or Phosphorylated Tau
As a protein clearing function, autophagy deficiency might be the cause of the aggregation and deposition of Aβ or hyperphosphorylation of tau in APs and NFTs (Pickford et al., 2008; Heckmann et al., 2019). Ca2+ signaling plays a crucial role in autophagy in various experimental models (Shaikh et al., 2016; Zhang et al., 2016). Logically, Ca2+ transporters are proposed to be involved in regulating autophagy. According to preliminary evidence, NMDARs on the cell membrane contribute to autophagy and the membrane potential in leukaemic megakaryoblasts (Nursalim, 2016). Specifically, exposure to low-dosage NMDA increases LC3 II production, which results in the degradation of GluR1, a subunit of AMPAR, in cultured rat hippocampal neurons (Shehata et al., 2012). Treatment with an antagonist of NMDAR, memantine, induces the NMDAR1-mediated autophagic cell death of T-98G cells (Yoon et al., 2017). In cultured hippocampal neurons, the NR2B antagonist Ro25-6981 markedly attenuates NMDA- and global ischaemia-induced activation of the autophagy pathway by disrupting the association of NR2B and Beclin1, resulting in cell death (Borsello et al., 2003; Liu and Zhao, 2013). In contrast, autophagy upregulates the expression of AMPAR subunits, including GluR1, GluR2, and GluR3, in oxygen- and glucose-deprived and reoxygenated injured neurons (Bao et al., 2017). These observations indicate the involvement of Ca2+ transporters located in the cell membranes in regulating autophagy. Similarly, VGCC induces Ca2+ influx to inhibit autophagy by activating calpains that cleave ATG5, an important factor for elongating autophagosomes, in H4 cells (Williams et al., 2008). As an atypical Ca2+ transporter in the cell membrane, APOE4 potentiates the effects of Aβ on the destabilization and permeabilization of lysosomal membranes, which results in impaired autophagy and the degradation of lysosomes in N2a cells (Ji et al., 2006; Nasiri-Ansari et al., 2021). In addition, rapamycin, an autophagy inducer, enhances mitochondrial autophagy and restores mitochondrial function in APOE4-expressing astrocytes (Schmukler et al., 2020). In astrocytes, APOE4 also impairs autophagy, resulting in attenuated clearance of Aβ (Simonovitch et al., 2016; Figure 1).
ER Stress Induces Autophagy by Modulating the Dyshomeostasis of Ca2+
In terms of intracellular Ca2+ stores, ER stress induces autophagy in propofol-stimulated C2C12 myoblast cells (Chen et al., 2018). In SK-N-SH cells, ER stress activates autophagy in UPR-stimulated SK-N-SH cells, which indicates its roles in AD (Nijholt et al., 2011). Specifically, polyglutamine induces LC3 conversion via ER stress, which initiates the onset of autophagy in C2C5 myoblast cells (Kouroku et al., 2007). Similarly, inducers of ER stress, including tunicamycin, DTT and MG132, concurrently decrease the activity of mTOR and increase the conversion of LC3 I to LC3 II in MEFs (Qin et al., 2010). Lithium induces autophagy by suppressing inositol monophosphatase, leading to the depletion of free inositol and InsP3 in SK-N-SH and COS-7 cells (Sarkar et al., 2005). This observation was also confirmed in lithium-treated IMPA1 knockout mice (Sade et al., 2016). In another study, Ca2+ was reported to be located downstream of InsP3R and mediated 2-aminoethoxydiphenyl borate (2-APB)-induced autophagy flux in neonatal rat ventricular myocytes (NRVMs) and HeLa cells (Wong et al., 2013). In addition, by inhibiting InsP3-mediated Ca2+ signaling, glucocorticoids induce autophagy in T lymphocytes (Harr et al., 2010). Blockade of InsP3R, the receptor of InsP3, restores autophagy and mitochondrial function in muscle fibers from WT and MDX mice (Valladares et al., 2018). InsP3R knockout upregulates the expression of autophagy markers compared to the WT controls (Cárdenas et al., 2010; Khan and Joseph, 2010). Researchers further emphasized the involvement of Ca2+ in autophagy by inducing autophagy through starvation and the activation of the InsP3R-mediated Ca2+ signaling pathway, as evidenced by the abolishment of LC3 lipidation and the formation of GFP-LC3 puncta in HeLa cells; these changes were blocked by the Ca2+ chelator BAPTA-AM and the InsP3R inhibitor xestospongin B (Cárdenas et al., 2010). In PC12 cells, isoflurane induced autophagy-dependent cell death via InsP3R-Ca2+-dependent mechanisms (Peng et al., 2011). Moreover, InsP3R-mediated transfer of Ca2+ from the ER to mitochondria is required to maintain the proper production of ATP, and Ca2+ blockade inhibits AMPK activity, leading to the suppression of autophagy in DT40 cells (Cárdenas et al., 2010; Lim et al., 2021a). Regarding the other Ca2+ transporters in ER membranes, RyR mediates the effects of propofol on inducing autophagy in cortical neuronal progenitor cells (Qiao et al., 2017). In primary cultured cortical neurons, RyR1 and RyR3 upregulation induced by insulin deprivation increase Ca2+ release from the ER, which increases the production of LC3II, an important autophagy marker (Edinger and Thompson, 2004; Chung et al., 2016). As an antagonist of RyRs, ryanodine stimulates autophagy by decreasing the cytosolic levels of Ca2+, leading to neuroprotection in CBE-N2a cells (Liou et al., 2016). By blocking RyR activity, dantrolene and an inhibitory dose of ryanodine reduce the conversion of LC3I to LC3II in HEK293 and C2C12 cells (Vervliet et al., 2017). Similarly, the downregulation of RyR2-mediated Ca2+ release decreases ATP production by suppressing mitochondrial metabolism, resulting in an increase in the autophagy-dependent death of rat neonatal cardiomyocytes (Pedrozo et al., 2013; McDaid et al., 2020). By depleting Ca2+ from the ER, SOCE exerts a biological effect on Ca2+ influx. In PC3 and DU145 cells, autophagic cell death was induced by resveratrol, which downregulated the expression of Stim1 and disrupted its association with TRPC1 and Orai1 (Selvaraj et al., 2016). The overexpression of Stim1 and Orai1 inhibits the effects of starvation- and rapamycin-induced autophagy on A7R5 rat arterial smooth muscle cells (Michiels et al., 2015). Moreover, caerulein promotes the interaction between Stim1 and Orai1, which activates CaN by inducing Ca2+ overload, leading to the expression of autophagy-related genes in mice with acute pancreatitis (Zhu et al., 2018). These observations revealed the involvement of ER Ca2+ stores in regulating autophagy (Figure 2).
Based on the aforementioned observations, InsP3R was found to connect mitochondria, potentially contributing to apoptosis and autophagy (Decuypere et al., 2011b). In Aβ-treated PC12 cells, moderate activation of autophagy regulates intracellular Ca2+ levels and the mitochondrial membrane potential (Xue et al., 2016). Reciprocally, mitochondrial fission-mediated Ca2+ signaling induces the expression of Stim1 and subsequent SOCE, which promoted autophagy through Ca2+/CAMKK/AMPK signaling cascades (Huang et al., 2017). Regarding Ca2+ transporters in mitochondria, VDAC recruits Parkin to defective mitochondria, resulting in the induction of mitochondrial autophagy in HEK293 cells (Sun et al., 2012). In addition, p53 is actively recruited to the outer membrane of mitochondria during nutrient deprivation, resulting in opening of the mPTP, an increase in the conversion of LC3BII to LC3BI, and the formation of LC3-GFP puncta in ventricular myocytes (Eydelnant et al., 2009; Xu H. X. et al., 2020).
Ca2+ Transporters on the Lysosomal Membranes Are Responsible for Regulating the Degradation of Aggregated Proteins
As the lysosome is the organelle responsible for degrading proteins, studies aiming to elucidate the roles of Ca2+ transporters located in lysosomes in regulating autophagy would be interesting. For example, Ca2+ stimulates lysosomal v-ATPase and mTORC1 pathways, which potentially contribute to the effects of orexin and hypocretin on autophagy in HEK293T cells (Wang et al., 2014). Rapamycin treatment inhibits mTOR activity by decreasing phosphorylation at two serine residues, leading to the induction of autophagy via a Ca2+-dependent mechanism (Onyenwoke et al., 2015). Furthermore, v-ATPase deficiency in Presenilin 1 (PS1) loss-of-function states causes deficits in lysosomes and autophagy, which contributes to abnormal cellular Ca2+ homeostasis (Lee et al., 2015). In addition, accumulating evidence is showing that the functional regulation of TRP channels contributes to Ca2+ signaling and subsequent autophagy initiation (Sukumaran et al., 2016). Transient receptor potential cation channel mucolipin subfamily member 1 (TRPML1) is a lysosomal Ca2+ channel, which can mediate the release of Ca2+ from lysosomes to cytoplasm. TRPML1 mutation increases the formation of autophagosomes, disrupts the fusion of autophagosomes and lysosomes, and induces the accumulation of p62 and insufficient removal of ubiquitinated proteins and/or defective mitochondria in fibroblasts from patients with mucolipidosis type IV (MLIV; Vergarajauregui et al., 2008; Nakamura et al., 2020). Under nutrient starvation conditions, TRPML1 upregulation is critical for increasing lysosomal proteolytic activity in COS-1 cells (Wang W. et al., 2015). Moreover, the overexpression of TRPML3/MCOLN3 induces autophagy in HeLa cells via a Ca2+-dependent mechanism (Kim et al., 2009). Similarly, both exogenous and endogenous Ca2+ modulate autophagy via different transporters (Table 5).
The Herbs Used as Food and Seasonings in Chinese Daily Life Potentially Contribute to Ad Treatment by Restoring The Ca2+ Concentration Through Effects on Its Transporters
As discussed above, Ca2+ overload plays important roles in aggravating AD via its transporters. In particular, Ca2+ overload perturbs the activities of the brain network, which increases the risk of AD and contributes causally to synaptic and cognitive deficits in hAPP mice. Since Ca2+ homeostasis is regulated by different transporters, transporters might be potential therapeutic targets for treating AD by modulating Ca2+ homeostasis. However, the outcome is not always consistent with our expectation. For instance, memantine, a noncompetitive NMDA antagonist, is an effective drug approved by the FDA for the treatment of AD. The VGCC inhibitor levetiracetam, an antiepileptic drug, exerts positive effects on patients with AD (Cumbo and Ligori, 2010; Vogl et al., 2012), whereas no beneficial therapeutic effect on AD was observed for the VGCC antagonist nilvadipine (Lawlor et al., 2018).
Although several FDA-approved chemical drugs are currently available for treating AD, the identification of new compounds targeting Ca2+ transporters to prevent, halt and reverse the dyshomeostasis of Ca2+ is urgently needed. We thereby summarized the drug candidates derived from herbs used as food or seasonings in Chinese daily life used to restore Ca2+ homeostasis in animals (Table 6). For example, asiatic acid from Centella asiatica reduces intracellular Ca2+ levels by inhibiting N- and P/Q-type calcium channels in the rat hippocampus (Lu et al., 2019). In rat cerebrocortical synaptosomes, silymarin derived from Silybum marianum similarly reduces intracellular Ca2+ concentrations by inhibiting N- and P/Q-type Ca2+ channels (Lu et al., 2020a). In addition, the I3C derivative [1(4-chloro-3-nitrobenzenesulfonyl)-1H-indol-3-yl]-methanol (CIM) from broccoli, cauliflower, and brussels sprouts inhibits Ca2+ influx by suppressing the activities of P/Q-type Ca2+ channels in rats (Lu et al., 2020b). In addition, numerous active compounds, such as uncarialin A, emodin, flavones, aconitine, patchouli alcohol (PA), coutareagenin, neferine, salvianolic acid B (Sal B), danshensu, tetrandrine, osthole, and hydroxy-safflor yellow A, derived from herbs, including Uncaria rhynchophylla, rhubarb, Acanthopanax senticosus (AS), Aconitum, Cablin, dandelion and Astragalus, plantule of Nelumbo nucifera, Salvia miltiorrhiza, Radix Salvia miltiorrhiza, Stephania tetrandra, Cnidium monnieri, and Carthamus tinctorius L., respectively, inhibit Ca2+ influx by deactivating Ca2+ transporters on the cell membrane, such as L-type Ca2+ channels, VDCC, G protein-coupled receptors, TRPCs, and TRPVs in different animal and cell models (Sun G. B. et al., 2014; Vierling et al., 2014; Zhou et al., 2014; Guan et al., 2015; Meng et al., 2016; Yang et al., 2016; Chen R. C. et al., 2017; Li et al., 2018; Yang J. et al., 2020; Yeh et al., 2020; Yu et al., 2020; Yun et al., 2020). Moreover, active compounds, including homoharringtonine, magnolol, polydatin (PD), and Ginkgo biloba extracts (EGb), derived from herbs, such as Cephalotaxus fortunei, magnolia tree, Polygonum cuspidatum, and Ginkgo biloba, respectively, modulate Ca2+ homeostasis by regulating the activities of transporters located in the ER through mechanism partially dependent on SOCE or mitochondria (Matsubara et al., 2005; Yang et al., 2013; Guo et al., 2014; Hsieh et al., 2018; Li et al., 2019). Although these herbs have not been used in clinical trials, all this evidence suggests that the herbs used as food and seasonings in Chinese daily life potentially contribute to treating AD by targeting Ca2+ transporters to restore Ca2+ concentrations (Table 6).
Conclusions
During the development and progression of AD, Ca2+ concentrations are increased in the cytosol of neuronal cells via transportation from the extracellular space and intracellular stores through transporter-dependent mechanisms. Ca2+ accumulation in neuronal cells induces the production and deposition of Aβ and hyperphosphorylated tau in APs and NFTs, leading to impaired learning ability in patients with AD. Moreover, transporters in the cell membrane, endoplasmic reticulum, mitochondria, and lysosomal membranes are critical for mediating the effects of Ca2+ on neuroinflammation, neuronal injury, neurogenesis, neurotoxicity, neuroprotection, autophagy, and synaptic plasticity, which contribute to the cognitive decline associated with AD (Figure 4). Based on these theoretical investigations, some bioactive components from Chinese herbal medicines have the potential to treat AD by targeting Ca2+ transporters. Moreover, Ca2+ transporters are progressively becoming new therapeutic targets for treating AD.
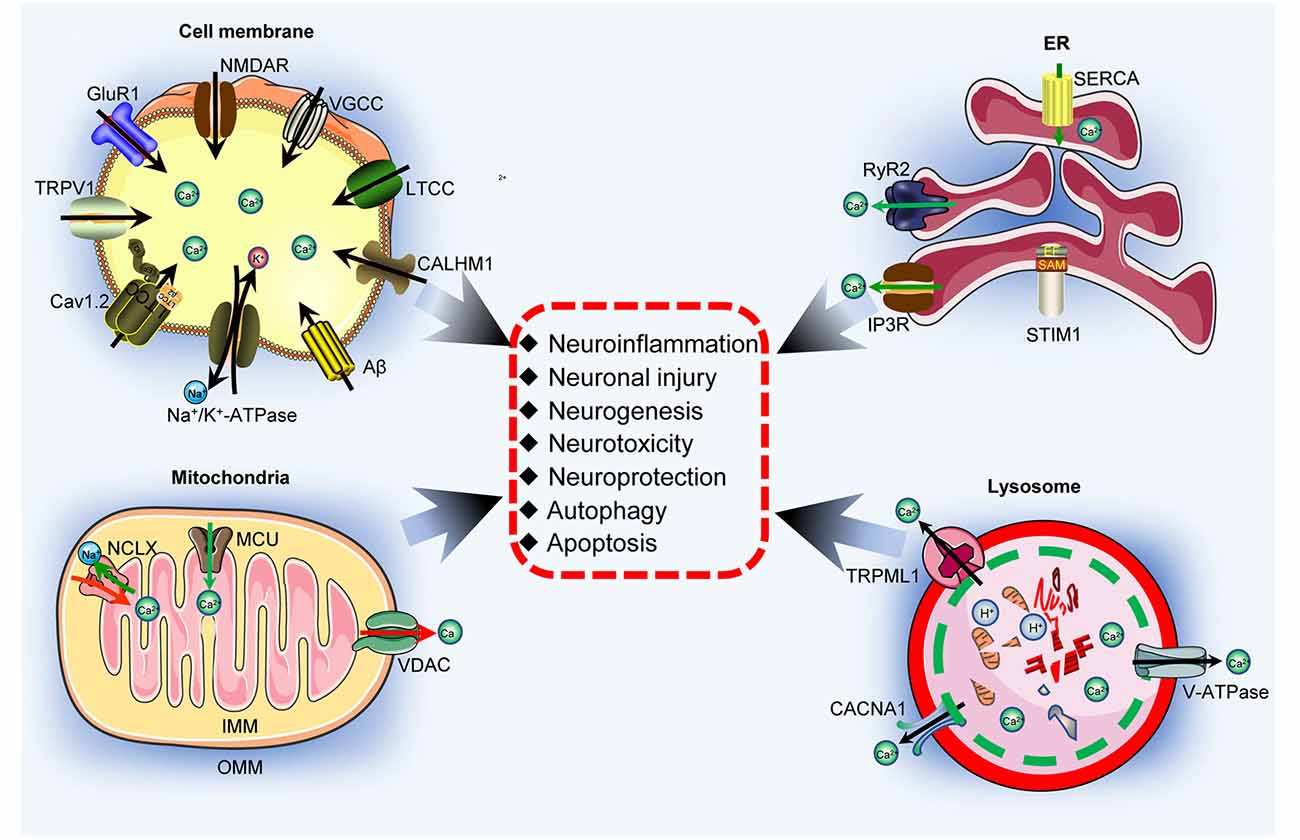
Figure 4. Ca2+ transporters are responsible for activating neuronal networks. Ca2+ transporters located in the cell membrane, ER, mitochondria, and lysosome are responsible for regulating neuroinflammation, neuronal injury, neurogenesis, neurotoxicity, neuroprotection, autophagy, and apoptosis.
Author Contributions
P-PG and L-LC contributed to conceptualizing and drafting the manuscript. YY contributed to summarizing the data presented in Table 6. PW contributed to conceptualizing, writing, reviewing, and editing the manuscript. All authors have agreed to publish the manuscript. All authors contributed to the article and approved the submitted version.
Funding
This work was supported in part or in whole by the National Natural Science Foundation of China (CN; 81771167 and 81870840).
Conflict of Interest
The authors declare that the research was conducted in the absence of any commercial or financial relationships that could be construed as a potential conflict of interest.
Publisher’s Note
All claims expressed in this article are solely those of the authors and do not necessarily represent those of their affiliated organizations, or those of the publisher, the editors and the reviewers. Any product that may be evaluated in this article, or claim that may be made by its manufacturer, is not guaranteed or endorsed by the publisher.
References
Ambrósio, A. F., Silva, A. P., Malva, J. O., Mesquita, J. F., Carvalho, A. P., and Carvalho, C. M. (2000). Role of desensitization of AMPA receptors on the neuronal viability and on the [Ca2+]i changes in cultured rat hippocampal neurons. Eur. J. Neurosci. 12, 2021–2031. doi: 10.1046/j.1460-9568.2000.00091.x
Arispe, N., Diaz, J., Durell, S. R., Shafrir, Y., and Guy, H. R. (2010). Polyhistidine peptide inhibitor of the Abeta calcium channel potently blocks the Abeta-induced calcium response in cells. Theoretical modeling suggests a cooperative binding process. Biochemistry 49, 7847–7853. doi: 10.1021/bi1006833
Bao, L., Li, R. H., Li, M., Jin, M. F., Li, G., Han, X., et al. (2017). Autophagy-regulated AMPAR subunit upregulation in in vitro oxygen glucose deprivation/reoxygenation-induced hippocampal injury. Brain Res. 1668, 65–71. doi: 10.1016/j.brainres.2017.05.019
Beattie, M. S., Ferguson, A. R., and Bresnahan, J. C. (2010). AMPA-receptor trafficking and injury-induced cell death. Eur. J. Neurosci. 32, 290–297. doi: 10.1111/j.1460-9568.2010.07343.x
Ben-Hail, D., and Shoshan-Barmatz, V. (2016). VDAC1-interacting anion transport inhibitors inhibit VDAC1 oligomerization and apoptosis. Biochim. Biophys. Acta 1863, 1612–1623. doi: 10.1016/j.bbamcr.2016.04.002
Berman, F. W., LePage, K. T., and Murray, T. F. (2002). Domoic acid neurotoxicity in cultured cerebellar granule neurons is controlled preferentially by the NMDA receptor Ca2+ influx pathway. Brain Res. 924, 20–29. doi: 10.1016/s0006-8993(01)03221-8
Bessou, M., Lopez, J., Gadet, R., Deygas, M., Popgeorgiev, N., Poncet, D., et al. (2020). The apoptosis inhibitor Bcl-xL controls breast cancer cell migration through mitochondria-dependent reactive oxygen species production. Oncogene 39, 3056–3074. doi: 10.1038/s41388-020-1212-9
Blackshaw, S., Sawa, A., Sharp, A. H., Ross, C. A., Snyder, S. H., and Khan, A. A. (2000). Type 3 inositol 1,4,5-trisphosphate receptor modulates cell death. FASEB J. 14, 1375–1379. doi: 10.1096/fj.14.10.1375
Boehmerle, W., Huehnchen, P., Lee, S. L. L., Harms, C., and Endres, M. (2018). TRPV4 inhibition prevents paclitaxel-induced neurotoxicity in preclinical models. Exp. Neurol. 306, 64–75. doi: 10.1016/j.expneurol.2018.04.014
Bollimuntha, S., Singh, B. B., Shavali, S., Sharma, S. K., and Ebadi, M. (2005). TRPC1-mediated inhibition of 1-methyl-4-phenylpyridinium ion neurotoxicity in human SH-SY5Y neuroblastoma cells. J. Biol. Chem. 280, 2132–2140. doi: 10.1074/jbc.M407384200
Borsello, T., Croquelois, K., Hornung, J. P., and Clarke, P. G. (2003). N-methyl-d-aspartate-triggered neuronal death in organotypic hippocampal cultures is endocytic, autophagic and mediated by the c-Jun N-terminal kinase pathway. Eur. J. Neurosci. 18, 473–485. doi: 10.1046/j.1460-9568.2003.02757.x
Brand-Schieber, E., and Werner, P. (2004). Calcium channel blockers ameliorate disease in a mouse model of multiple sclerosis. Exp. Neurol. 189, 5–9. doi: 10.1016/j.expneurol.2004.05.023
Brookhouser, N., Raman, S., Frisch, C., Srinivasan, G., and Brafman, D. A. (2021). APOE2 mitigates disease-related phenotypes in an isogenic hiPSC-based model of Alzheimer’s disease. Mol. Psychiatry [Online ahead of print]. doi: 10.1038/s41380-021-01076-3
Brown, G. C., and Neher, J. J. (2014). Microglial phagocytosis of live neurons. Nat. Rev. Neurosci. 15, 209–216. doi: 10.1038/nrn3710
Brustein, E., Côté, S., Ghislain, J., and Drapeau, P. (2013). Spontaneous glycine-induced calcium transients in spinal cord progenitors promote neurogenesis. Dev. Neurobiol. 73, 168–175. doi: 10.1002/dneu.22050
Bull, R., Finkelstein, J. P., Gálvez, J., Sánchez, G., Donoso, P., Behrens, M. I., et al. (2008). Ischemia enhances activation by Ca2+ and redox modification of ryanodine receptor channels from rat brain cortex. J. Neurosci. 28, 9463–9472. doi: 10.1523/JNEUROSCI.2286-08.2008
Bullock, R. (2006). Efficacy and safety of memantine in moderate-to-severe Alzheimer disease: the evidence to date. Alzheimer Dis. Assoc. Disord. 20, 23–29. doi: 10.1097/01.wad.0000201847.29836.a5
Bursztajn, S., Falls, W. A., Berman, S. A., and Friedman, M. J. (2007). Cell proliferation in the brains of NMDAR NR1 transgenic mice. Brain Res. 1172, 10–20. doi: 10.1016/j.brainres.2007.07.045
Cai, F., Liu, J., Li, C., and Wang, J. (2015). Intracellular calcium plays a critical role in the microcystin-LR-elicited neurotoxicity through PLC/IP3 pathway. Int. J. Toxicol. 34, 551–558. doi: 10.1177/1091581815606352
Cai, Y., Zhang, X., Zhou, X., Wu, X., Li, Y., Yao, J., et al. (2017). Nicotine suppresses the neurotoxicity by MPP(+)/MPTP through activating α7nAChR/PI3K/Trx-1 and suppressing ER stress. Neurotoxicology 59, 49–55. doi: 10.1016/j.neuro.2017.01.002
Calvo-Rodriguez, M., Hernando-Pérez, E., López-Vázquez, S., Núñez, J., Villalobos, C., and Núñez, L. (2020). Remodeling of intracellular Ca(2+) homeostasis in rat hippocampal neurons aged in vitro. Int. J. Mol. Sci. 21:1549. doi: 10.3390/ijms21041549
Cao, L. L., Guan, P. P., Liang, Y. Y., Huang, X. S., and Wang, P. (2019). Calcium ions stimulate the hyperphosphorylation of tau by activating microsomal prostaglandin E synthase 1. Front. Aging Neurosci. 11:108. doi: 10.3389/fnagi.2019.00108
Cao, X., and Südhof, T. C. (2001). A transcriptionally [correction of transcriptively] active complex of APP with Fe65 and histone acetyltransferase Tip60. Science 293, 115–120. doi: 10.1126/science.1058783
Cárdenas, C., Miller, R. A., Smith, I., Bui, T., Molgó, J., Müller, M., et al. (2010). Essential regulation of cell bioenergetics by constitutive InsP3 receptor Ca2+ transfer to mitochondria. Cell 142, 270–283. doi: 10.1016/j.cell.2010.06.007
Cardozo, A. K., Ortis, F., Storling, J., Feng, Y. M., Rasschaert, J., Tonnesen, M., et al. (2005). Cytokines downregulate the sarcoendoplasmic reticulum pump Ca2+ ATPase 2b and deplete endoplasmic reticulum Ca2+, leading to induction of endoplasmic reticulum stress in pancreatic beta-cells. Diabetes 54, 452–461. doi: 10.2337/diabetes.54.2.452
Chao, C. C., Huang, C. C., Lu, D. Y., Wong, K. L., Chen, Y. R., Cheng, T. H., et al. (2012). Ca2+ store depletion and endoplasmic reticulum stress are involved in P2X7 receptor-mediated neurotoxicity in differentiated NG108–15 cells. J. Cell. Biochem. 113, 1377–1385. doi: 10.1002/jcb.24010
Chen, S., Averett, N. T., Manelli, A., Ladu, M. J., May, W., and Ard, M. D. (2005). Isoform-specific effects of apolipoprotein E on secretion of inflammatory mediators in adult rat microglia. J. Alzheimers Dis. 7, 25–35. doi: 10.3233/jad-2005-7104
Chen, T., Dai, S. H., Jiang, Z. Q., Luo, P., Jiang, X. F., Fei, Z., et al. (2017). The AMPAR antagonist perampanel attenuates traumatic brain injury through anti-oxidative and anti-inflammatory activity. Cell. Mol. Neurobiol. 37, 43–52. doi: 10.1007/s10571-016-0341-8
Chen, H., Gao, W., Yang, Y., Guo, S., Wang, H., Wang, W., et al. (2014). Inhibition of VDAC1 prevents Ca2-mediated oxidative stress and apoptosis induced by 5-aminolevulinic acid mediated sonodynamic therapy in THP-1 macrophages. Apoptosis 19, 1712–1726. doi: 10.1007/s10495-014-1045-5
Chen, J., Li, Z., Hatcher, J. T., Chen, Q. H., Chen, L., Wurster, R. D., et al. (2017). Deletion of TRPC6 attenuates NMDA receptor-mediated Ca(2+) entry and Ca(2+)-induced neurotoxicity following cerebral ischemia and oxygen-glucose deprivation. Front. Neurosci. 11:138. doi: 10.3389/fnins.2017.00138
Chen, X., Li, L. Y., Jiang, J. L., Li, K., Su, Z. B., Zhang, F. Q., et al. (2018). Propofol elicits autophagy via endoplasmic reticulum stress and calcium exchange in C2C12 myoblast cell line. PLoS One 13:e0197934. doi: 10.1371/journal.pone.0197934
Chen, R. C., Sun, G. B., Ye, J. X., Wang, J., Zhang, M. D., and Sun, X. B. (2017). Salvianolic acid B attenuates doxorubicin-induced ER stress by inhibiting TRPC3 and TRPC6 mediated Ca(2+) overload in rat cardiomyocytes. Toxicol. Lett. 276, 21–30. doi: 10.1016/j.toxlet.2017.04.010
Cheng, A., Kawahata, I., and Fukunaga, K. (2020). Fatty acid binding protein 5 mediates cell death by psychosine exposure through mitochondrial macropores formation in oligodendrocytes. Biomedicines 8:635. doi: 10.3390/biomedicines8120635
Cheng, L., Muroi, M., Cao, S., Bian, L., Osada, H., Xiang, L., et al. (2019). 3β,23,28-Trihydroxy-12-oleanene 3β-caffeate from desmodium sambuense-induced neurogenesis in PC12 cells mediated by ER stress and BDNF-TrkB signaling pathways. Mol. Pharm. 16, 1423–1432. doi: 10.1021/acs.molpharmaceut.8b00939
Choi, I. Y., Lim, J. H., Kim, C., Song, H. Y., Ju, C., and Kim, W. K. (2013). 4-hydroxy-2(E)-nonenal facilitates nmda-induced neurotoxicity via triggering mitochondrial permeability transition pore opening and mitochondrial calcium overload. Exp. Neurobiol. 22, 200–207. doi: 10.5607/en.2013.22.3.200
Chung, K. M., Jeong, E. J., Park, H., An, H. K., and Yu, S. W. (2016). Mediation of autophagic cell death by type 3 ryanodine receptor (RyR3) in adult hippocampal neural stem cells. Front. Cell. Neurosci. 10:116. doi: 10.3389/fncel.2016.00116
Coen, K., Flannagan, R. S., Baron, S., Carraro-Lacroix, L. R., Wang, D., Vermeire, W., et al. (2012). Lysosomal calcium homeostasis defects, not proton pump defects, cause endo-lysosomal dysfunction in PSEN-deficient cells. J. Cell. Biol. 198, 23–35. doi: 10.1083/jcb.201201076
Cui, R., Yan, L., Luo, Z., Guo, X., and Yan, M. (2015). Blockade of store-operated calcium entry alleviates ethanol-induced hepatotoxicity via inhibiting apoptosis. Toxicol. Appl. Pharmacol. 287, 52–66. doi: 10.1016/j.taap.2015.05.014
Cumbo, E., and Ligori, L. D. (2010). Levetiracetam, lamotrigine and phenobarbital in patients with epileptic seizures and Alzheimer’s disease. Epilepsy Behav. 17, 461–466. doi: 10.1016/j.yebeh.2010.01.015
Dafnis, I., Argyri, L., and Chroni, A. (2018). Amyloid-peptide β 42 enhances the oligomerization and neurotoxicity of apoE4: The C-terminal residues Leu279, Lys282 and Gln284 modulate the structural and functional properties of apoE4. Neuroscience 394, 144–155. doi: 10.1016/j.neuroscience.2018.10.026
D’Ascenzo, M., Piacentini, R., Casalbore, P., Budoni, M., Pallini, R., Azzena, G. B., et al. (2006). Role of L-type Ca2+ channels in neural stem/progenitor cell differentiation. Eur. J. Neurosci. 23, 935–944. doi: 10.1111/j.1460-9568.2006.04628.x
Daschil, N., Obermair, G. J., Flucher, B. E., Stefanova, N., Hutter-Paier, B., Windisch, M., et al. (2013). CaV1.2 calcium channel expression in reactive astrocytes is associated with the formation of amyloid-β plaques in an Alzheimer’s disease mouse model. J. Alzheimers Dis. 37, 439–451. doi: 10.3233/JAD-130560
Deb, B. K., Chakraborty, P., Gopurappilly, R., and Hasan, G. (2020). SEPT7 regulates Ca(2+) entry through Orai channels in human neural progenitor cells and neurons. Cell Calcium 90:102252. doi: 10.1016/j.ceca.2020.102252
Decuypere, J. P., Bultynck, G., and Parys, J. B. (2011a). A dual role for Ca(2+) in autophagy regulation. Cell Calcium 50, 242–250. doi: 10.1016/j.ceca.2011.04.001
Decuypere, J. P., Monaco, G., Bultynck, G., Missiaen, L., De Smedt, H., and Parys, J. B. (2011b). The IP(3) receptor-mitochondria connection in apoptosis and autophagy. Biochim. Biophys. Acta 1813, 1003–1013. doi: 10.1016/j.bbamcr.2010.11.023
Deep, S. N., Mitra, S., Rajagopal, S., Paul, S., and Poddar, R. (2019). GluN2A-NMDA receptor-mediated sustained Ca(2+) influx leads to homocysteine-induced neuronal cell death. J. Biol. Chem. 294, 11154–11165. doi: 10.1074/jbc.RA119.008820
Dolga, A. M., Letsche, T., Gold, M., Doti, N., Bacher, M., Chiamvimonvat, N., et al. (2012). Activation of KCNN3/SK3/K(Ca)2.3 channels attenuates enhanced calcium influx and inflammatory cytokine production in activated microglia. Glia 60, 2050–2064. doi: 10.1002/glia.22419
Domenichini, F., Terrié, E., Arnault, P., Harnois, T., Magaud, C., Bois, P., et al. (2018). Store-operated calcium entries control neural stem cell self-renewal in the adult brain subventricular zone. Stem Cells 36, 761–774. doi: 10.1002/stem.2786
Dong, Y., Kalueff, A. V., and Song, C. (2017). N-methyl-d-aspartate receptor-mediated calcium overload and endoplasmic reticulum stress are involved in interleukin-1beta-induced neuronal apoptosis in rat hippocampus. J. Neuroimmunol. 307, 7–13. doi: 10.1016/j.jneuroim.2017.03.005
Drouet, B., Fifre, A., Pinçon-Raymond, M., Vandekerckhove, J., Rosseneu, M., Guéant, J. L., et al. (2001). ApoE protects cortical neurones against neurotoxicity induced by the non-fibrillar C-terminal domain of the amyloid-beta peptide. J. Neurochem. 76, 117–127. doi: 10.1046/j.1471-4159.2001.00047.x
Du, H., Guo, L., Fang, F., Chen, D., Sosunov, A. A., McKhann, G. M., et al. (2008). Cyclophilin D deficiency attenuates mitochondrial and neuronal perturbation and ameliorates learning and memory in Alzheimer’s disease. Nat. Med. 14, 1097–1105. doi: 10.1038/nm.1868
Du, L. L., Wang, L., Yang, X. F., Wang, P., Li, X. H., Chai, D. M., et al. (2017). Transient receptor potential-canonical 1 is essential for environmental enrichment-induced cognitive enhancement and neurogenesis. Mol. Neurobiol. 54, 1992–2002. doi: 10.1007/s12035-016-9758-9
Durell, S. R., Guy, H. R., Arispe, N., Rojas, E., and Pollard, H. B. (1994). Theoretical models of the ion channel structure of amyloid beta-protein. Biophys. J. 67, 2137–2145. doi: 10.1016/S0006-3495(94)80717-9
Edinger, A. L., and Thompson, C. B. (2004). Death by design: apoptosis, necrosis and autophagy. Curr Opin Cell Biol 16, 663–669. doi: 10.1016/j.ceb.2004.09.011
Elgh, E., Lindqvist Astot, A., Fagerlund, M., Eriksson, S., Olsson, T., and Näsman, B. (2006). Cognitive dysfunction, hippocampal atrophy and glucocorticoid feedback in Alzheimer’s disease. Biol. Psychiatry 59, 155–161. doi: 10.1016/j.biopsych.2005.06.017
Ertilav, K., Nazıroğlu, M., Ataizi, Z. S., and Yıldızhan, K. (2021). Melatonin and selenium suppress docetaxel-induced TRPV1 activation, neuropathic pain and oxidative neurotoxicity in mice. Biol. Trace Elem. Res. 199, 1469–1487. doi: 10.1007/s12011-020-02250-4
Espinosa-Parrilla, J. F., Martínez-Moreno, M., Gasull, X., Mahy, N., and Rodríguez, M. J. (2015). The L-type voltage-gated calcium channel modulates microglial pro-inflammatory activity. Mol. Cell. Neurosci. 64, 104–115. doi: 10.1016/j.mcn.2014.12.004
Etcheberrigaray, R., Hirashima, N., Nee, L., Prince, J., Govoni, S., Racchi, M., et al. (1998). Calcium responses in fibroblasts from asymptomatic members of Alzheimer’s disease families. Neurobiol. Dis. 5, 37–45. doi: 10.1006/nbdi.1998.0176
Evrard, Y. A., Mohammad-Zadeh, L., and Holton, B. (2004). Alterations in Ca2+-dependent and cAMP-dependent signaling pathways affect neurogenesis and melanogenesis of quail neural crest cells in vitro. Dev. Genes Evol. 214, 193–199. doi: 10.1007/s00427-004-0395-3
Eydelnant, I. A., Zhang, T., Bednarczyk, J., and Gang, H. Y. (2009). Abstract 3994: recruitment of p53 to mitochondrial VDAC1 triggers autophagy of ventricular myocytes during metabolic stress. Circulation 120:S902.
Fan, H., Gao, J., Wang, W., Li, X., Xu, T., and Yin, X. (2012). Expression of NMDA receptor and its effect on cell proliferation in the subventricular zone of neonatal rat brain. Cell Biochem. Biophys. 62, 305–316. doi: 10.1007/s12013-011-9302-5
Faure, A. V., Grunwald, D., Moutin, M. J., Hilly, M., Mauger, J. P., Marty, I., et al. (2001). Developmental expression of the calcium release channels during early neurogenesis of the mouse cerebral cortex. Eur. J. Neurosci. 14, 1613–1622. doi: 10.1046/j.0953-816x.2001.01786.x
Ferguson, A. R., Christensen, R. N., Gensel, J. C., Miller, B. A., Sun, F., Beattie, E. C., et al. (2008). Cell death after spinal cord injury is exacerbated by rapid TNF alpha-induced trafficking of GluR2-lacking AMPARs to the plasma membrane. J. Neurosci. 28, 11391–11400. doi: 10.1523/JNEUROSCI.3708-08.2008
Fernandez, A. M., Fernandez, S., Carrero, P., Garcia-Garcia, M., and Torres-Aleman, I. (2007). Calcineurin in reactive astrocytes plays a key role in the interplay between proinflammatory and anti-inflammatory signals. J. Neurosci. 27, 8745–8756. doi: 10.1523/JNEUROSCI.1002-07.2007
Fernandez-Echevarria, C., Díaz, M., Ferrer, I., Canerina-Amaro, A., and Marin, R. (2014). Aβ promotes VDAC1 channel dephosphorylation in neuronal lipid rafts. Relevance to the mechanisms of neurotoxicity in Alzheimer’s disease. Neuroscience 278, 354–366. doi: 10.1016/j.neuroscience.2014.07.079
Fernandez-Funez, P., Casas-Tinto, S., Zhang, Y., and Rincon-Limas, D. (2010). P4-078: Xbp1s prevents amyloid-β neurotoxicity by regulating ryanodine Ca2+ channels. Alzheimer’s Dement. 6, e45–e45. doi: 10.1016/j.jalz.2010.08.139
Ferreiro, E., Oliveira, C. R., and Pereira, C. (2004). Involvement of endoplasmic reticulum Ca2+ release through ryanodine and inositol 1,4,5-triphosphate receptors in the neurotoxic effects induced by the amyloid-beta peptide. J. Neurosci. Res. 76, 872–880. doi: 10.1002/jnr.20135
Ferreiro, E., Resende, R., Costa, R., Oliveira, C. R., and Pereira, C. M. (2006). An endoplasmic-reticulum-specific apoptotic pathway is involved in prion and amyloid-beta peptides neurotoxicity. Neurobiol. Dis. 23, 669–678. doi: 10.1016/j.nbd.2006.05.011
Fiorio Pla, A., Maric, D., Brazer, S. C., Giacobini, P., Liu, X., Chang, Y. H., et al. (2005). Canonical transient receptor potential 1 plays a role in basic fibroblast growth factor (bFGF)/FGF receptor-1-induced Ca2+ entry and embryonic rat neural stem cell proliferation. J. Neurosci. 25, 2687–2701. doi: 10.1523/JNEUROSCI.0951-04.2005
Flourakis, M., Lehen’kyi, V., Beck, B., Raphaël, M., Vandenberghe, M., Abeele, F. V., et al. (2010). Orai1 contributes to the establishment of an apoptosis-resistant phenotype in prostate cancer cells. Cell Death Dis. 1:e75. doi: 10.1038/cddis.2010.52
Fonfria, E., Marshall, I. C., Boyfield, I., Skaper, S. D., Hughes, J. P., Owen, D. E., et al. (2005). Amyloid beta-peptide(1–42) and hydrogen peroxide-induced toxicity are mediated by TRPM2 in rat primary striatal cultures. J. Neurochem. 95, 715–723. doi: 10.1111/j.1471-4159.2005.03396.x
Fonfria, E., Mattei, C., Hill, K., Brown, J. T., Randall, A., Benham, C. D., et al. (2006). TRPM2 is elevated in the tMCAO stroke model, transcriptionally regulated and functionally expressed in C13 microglia. J. Recept. Signal Transduct. Res. 26, 179–198. doi: 10.1080/10799890600637522
Franciosi, S., Choi, H. B., Kim, S. U., and McLarnon, J. G. (2002). Interferon-gamma acutely induces calcium influx in human microglia. J. Neurosci. Res. 69, 607–613. doi: 10.1002/jnr.10331
Frandsen, A., and Schousboe, A. (1991). Dantrolene prevents glutamate cytotoxicity and Ca2+ release from intracellular stores in cultured cerebral cortical neurons. J. Neurochem. 56, 1075–1078. doi: 10.1111/j.1471-4159.1991.tb02031.x
Furman, J. L., Sama, D. M., Gant, J. C., Beckett, T. L., Murphy, M. P., Bachstetter, A. D., et al. (2012). Targeting astrocytes ameliorates neurologic changes in a mouse model of Alzheimer’s disease. J. Neurosci. 32, 16129–16140. doi: 10.1523/JNEUROSCI.2323-12.2012
Furukawa, K., and Mattson, M. P. (1998). The transcription factor NF-kappaB mediates increases in calcium currents and decreases in NMDA- and AMPA/kainate-induced currents induced by tumor necrosis factor-alpha in hippocampal neurons. J. Neurochem. 70, 1876–1886. doi: 10.1046/j.1471-4159.1998.70051876.x
Ganguly, P., Honeycutt, J. A., Rowe, J. R., Demaestri, C., and Brenhouse, H. C. (2019). Effects of early life stress on cocaine conditioning and AMPA receptor composition are sex-specific and driven by TNF. Brain Behav. Immun. 78, 41–51. doi: 10.1016/j.bbi.2019.01.006
Garrosa, J., Paredes, I., Marambaud, P., López, M. G., and Cano-Abad, M. F. (2020). Molecular and pharmacological modulation of CALHM1 promote neuroprotection against oxygen and glucose deprivation in a model of hippocampal slices. Cells 9:664. doi: 10.3390/cells9030664
Geffin, R., Martinez, R., de Las Pozas, A., Issac, B., and McCarthy, M. (2017). Apolipoprotein E4 suppresses neuronal-specific gene expression in maturing neuronal progenitor cells exposed to HIV. J. Neuroimmune. Pharmacol. 12, 462–483. doi: 10.1007/s11481-017-9734-9
Gerace, E., Ilari, A., Caffino, L., Buonvicino, D., Lana, D., Ugolini, F., et al. (2021). Ethanol neurotoxicity is mediated by changes in expression, surface localization and functional properties of glutamate AMPA receptors. J. Neurochem. 157, 2106–2118. doi: 10.1111/jnc.15223
Giarratana, A. O., Zheng, C., Reddi, S., Teng, S. L., Berger, D., Adler, D., et al. (2020). APOE4 genetic polymorphism results in impaired recovery in a repeated mild traumatic brain injury model and treatment with Bryostatin-1 improves outcomes. Sci. Rep. 10:19919. doi: 10.1038/s41598-020-76849-x
Gkouveris, I., Nikitakis, N. G., Aseervatham, J., and Ogbureke, K. U. E. (2018). Interferon γ suppresses dentin sialophosphoprotein in oral squamous cell carcinoma cells resulting in antitumor effects, via modulation of the endoplasmic reticulum response. Int. J. Oncol. 53, 2423–2432. doi: 10.3892/ijo.2018.4590
Goghari, V., Franciosi, S., Kim, S. U., Lee, Y. B., and McLarnon, J. G. (2000). Acute application of interleukin-1beta induces Ca(2+) responses in human microglia. Neurosci. Lett. 281, 83–86. doi: 10.1016/s0304-3940(00)00824-7
Goswami, P., Afjal, M. A., Akhter, J., Mangla, A., Khan, J., Parvez, S., et al. (2020). Involvement of endoplasmic reticulum stress in amyloid β ((1–42))-induced Alzheimer’s like neuropathological process in rat brain. Brain Res. Bull. 165, 108–117. doi: 10.1016/j.brainresbull.2020.09.022
Grotemeier, A., Alers, S., Pfisterer, S. G., Paasch, F., Daubrawa, M., Dieterle, A., et al. (2010). AMPK-independent induction of autophagy by cytosolic Ca2+ increase. Cell. Signal. 22, 914–925. doi: 10.1016/j.cellsig.2010.01.015
Gu, C., Li, L., Huang, Y., Q25an, D., Liu, W., Zhang, C., et al. (2020). Salidroside ameliorates mitochondria-dependent neuronal apoptosis after spinal cord ischemia-reperfusion injury partially through inhibiting oxidative stress and promoting mitophagy. Oxid. Med. Cell. Longev. 2020:3549704. doi: 10.1155/2020/3549704
Guan, P. P., Cao, L. L., and Wang, P. (2021). Elevating the levels of calcium ions exacerbate Alzheimer’s disease via inducing the production and aggregation of β-amyloid protein and phosphorylated Tau. Int. J. Mol. Sci. 22:5900. doi: 10.3390/ijms22115900
Guan, S., Ma, J., Chu, X., Gao, Y., Zhang, Y., Zhang, X., et al. (2015). Effects of total flavones from Acanthopanax senticosus on L-type calcium channels, calcium transient and contractility in rat ventricular myocytes. Phytother. Res. 29, 533–539. doi: 10.1002/ptr.5278
Guo, Y., Han, S., Cao, J., Liu, Q., and Zhang, T. (2014). Screening of allergic components mediated by H(1)R in homoharringtonine injection through H(1)R/CMC-HPLC/MS. Biomed. Chromatogr. 28, 1607–1614. doi: 10.1002/bmc.3188
Güzel, M., Nazıroğlu, M., Akpınar, O., and Çınar, R. (2021). Interferon gamma-mediated oxidative stress induces apoptosis, neuroinflammation, zinc ion influx, and TRPM2 channel activation in neuronal cell line: modulator role of curcumin. Inflammation 44, 1878–1894. doi: 10.1007/s10753-021-01465-4
Han, X. J., Hu, Y. Y., Yang, Z. J., Jiang, L. P., Shi, S. L., Li, Y. R., et al. (2017). Amyloid β-42 induces neuronal apoptosis by targeting mitochondria. Mol. Med. Rep. 16, 4521–4528. doi: 10.3892/mmr.2017.7203
Hao, B., Lu, Y., Wang, Q., Guo, W., Cheung, K. H., and Yue, J. (2014). Role of STIM1 in survival and neural differentiation of mouse embryonic stem cells independent of Orai1-mediated Ca2+ entry. Stem Cell Res. 12, 452–466. doi: 10.1016/j.scr.2013.12.005
Harr, M. W., McColl, K. S., Zhong, F., Molitoris, J. K., and Distelhorst, C. W. (2010). Glucocorticoids downregulate Fyn and inhibit IP(3)-mediated calcium signaling to promote autophagy in T lymphocytes. Autophagy 6, 912–921. doi: 10.4161/auto.6.7.13290
Heckmann, B. L., Teubner, B. J. W., Tummers, B., Boada-Romero, E., Harris, L., Yang, M., et al. (2019). LC3-associated endocytosis facilitates β-amyloid clearance and mitigates neurodegeneration in murine Alzheimer’s disease. Cell 178, 536–551.e514. doi: 10.1016/j.cell.2019.05.056
Holland, E. B., and Pessah, I. N. (2021). Non-dioxin-like polychlorinated biphenyl neurotoxic equivalents found in environmental and human samples. Regul. Toxicol. Pharmacol. 120:104842. doi: 10.1016/j.yrtph.2020.104842
Hong, S. H., Choi, H. B., Kim, S. U., and McLarnon, J. G. (2006). Mitochondrial ligand inhibits store-operated calcium influx and COX-2 production in human microglia. J. Neurosci. Res. 83, 1293–1298. doi: 10.1002/jnr.20829
Hong, S., Kim, A., and Kernie, S. (2013). Apoe4 impairs injury-induced neurogenesis. Critical Care Med. 41, A39–A40. doi: 10.1097/01.ccm.0000439329.97813.61
Hopp, S. C., Royer, S. E., D’Angelo, H. M., Kaercher, R. M., Fisher, D. A., and Wenk, G. L. (2015). Differential neuroprotective and anti-inflammatory effects of L-type voltage dependent calcium channel and ryanodine receptor antagonists in the substantia nigra and locus coeruleus. J. Neuroimmune Pharmacol. 10, 35–44. doi: 10.1007/s11481-014-9568-7
Hou, Y., Ghosh, P., Wan, R., Ouyang, X., Cheng, H., Mattson, M. P., et al. (2014). Permeability transition pore-mediated mitochondrial superoxide flashes mediate an early inhibitory effect of amyloid beta1–42 on neural progenitor cell proliferation. Neurobiol. Aging 35, 975–989. doi: 10.1016/j.neurobiolaging.2013.11.002
Hou, P. F., Liu, Z. H., Li, N., Cheng, W. J., and Guo, S. W. (2015). Knockdown of STIM1 improves neuronal survival after traumatic neuronal injury through regulating mGluR1-dependent Ca(2+) signaling in mouse cortical neurons. Cell. Mol. Neurobiol. 35, 283–292. doi: 10.1007/s10571-014-0123-0
Hou, Y., Mattson, M. P., and Cheng, A. (2013). Permeability transition pore-mediated mitochondrial superoxide flashes regulate cortical neural progenitor differentiation. PLoS One 8:e76721. doi: 10.1371/journal.pone.0076721
Høyer-Hansen, M., Bastholm, L., Szyniarowski, P., Campanella, M., Szabadkai, G., Farkas, T., et al. (2007). Control of macroautophagy by calcium, calmodulin-dependent kinase kinase-beta and Bcl-2. Mol. Cell 25, 193–205. doi: 10.1016/j.molcel.2006.12.009
Hsieh, S. F., Chou, C. T., Liang, W. Z., Kuo, C. C., Wang, J. L., Hao, L. J., et al. (2018). The effect of magnolol on Ca(2+) homeostasis and its related physiology in human oral cancer cells. Arch. Oral Biol. 89, 49–54. doi: 10.1016/j.archoralbio.2018.02.006
Huang, Q., Cao, H., Zhan, L., Sun, X., Wang, G., Li, J., et al. (2017). Mitochondrial fission forms a positive feedback loop with cytosolic calcium signaling pathway to promote autophagy in hepatocellular carcinoma cells. Cancer Lett 403, 108–118. doi: 10.1016/j.canlet.2017.05.034
Huang, Y., Smith, D. E., Ibáñez-Sandoval, O., Sims, J. E., and Friedman, W. J. (2011). Neuron-specific effects of interleukin-1β are mediated by a novel isoform of the IL-1 receptor accessory protein. J. Neurosci. 31, 18048–18059. doi: 10.1523/JNEUROSCI.4067-11.2011
Hudecova, S., Markova, J., Simko, V., Csaderova, L., Stracina, T., Sirova, M., et al. (2016). Sulforaphane-induced apoptosis involves the type 1 IP3 receptor. Oncotarget 7, 61403–61418. doi: 10.18632/oncotarget.8968
Hudry, E., Dashkoff, J., Roe, A. D., Takeda, S., Koffie, R. M., Hashimoto, T., et al. (2013). Gene transfer of human Apoe isoforms results in differential modulation of amyloid deposition and neurotoxicity in mouse brain. Sci. Transl. Med. 5:212ra161. doi: 10.1126/scitranslmed.3007000
Jang, H., Ma, B., Lal, R., and Nussinov, R. (2008). Models of toxic beta-sheet channels of protegrin-1 suggest a common subunit organization motif shared with toxic alzheimer beta-amyloid ion channels. Biophys. J. 95, 4631–4642. doi: 10.1529/biophysj.108.134551
Ji, Y., Hu, Y., Ren, J., Khanna, R., Yao, Y., Chen, Y., et al. (2019). CRMP2-derived peptide ST2–104 (R9-CBD3) protects SH-SY5Y neuroblastoma cells against Aβ(25–35)-induced neurotoxicity by inhibiting the pCRMP2/NMDAR2B signaling pathway. Chem. Biol. Interact 305, 28–39. doi: 10.1016/j.cbi.2019.03.005
Ji, Z. S., Müllendorff, K., Cheng, I. H., Miranda, R. D., Huang, Y., and Mahley, R. W. (2006). Reactivity of apolipoprotein E4 and amyloid beta peptide: lysosomal stability and neurodegeneration. J. Biol. Chem. 281, 2683–2692. doi: 10.1074/jbc.M506646200
Jiang, L., Zhong, J., Dou, X., Cheng, C., Huang, Z., and Sun, X. (2015). Effects of ApoE on intracellular calcium levels and apoptosis of neurons after mechanical injury. Neuroscience 301, 375–383. doi: 10.1016/j.neuroscience.2015.06.005
Joseph, J. D., Peng, Y., Mak, D. O., Cheung, K. H., Vais, H., Foskett, J. K., et al. (2014). General anesthetic isoflurane modulates inositol 1,4,5-trisphosphate receptor calcium channel opening. Anesthesiology 121, 528–537. doi: 10.1097/ALN.0000000000000316
Ju, Y., Ge, J., Ren, X., Zhu, X., Xue, Z., Feng, Y., et al. (2015). Cav1.2 of L-type calcium channel is a key factor for the differentiation of dental pulp stem cells. J. Endod. 41, 1048–1055. doi: 10.1016/j.joen.2015.01.009
Kakae, M., Miyanohara, J., Morishima, M., Nagayasu, K., Mori, Y., Shirakawa, H., et al. (2019). Pathophysiological role of TRPM2 in age-related cognitive impairment in mice. Neuroscience 408, 204–213. doi: 10.1016/j.neuroscience.2019.04.012
Kaneko, S., Kawakami, S., Hara, Y., Wakamori, M., Itoh, E., Minami, T., et al. (2006). A critical role of TRPM2 in neuronal cell death by hydrogen peroxide. J. Pharmacol. Sci. 101, 66–76. doi: 10.1254/jphs.fp0060128
Kawada, K., Iekumo, T., Saito, R., Kaneko, M., Mimori, S., Nomura, Y., et al. (2014). Aberrant neuronal differentiation and inhibition of dendrite outgrowth resulting from endoplasmic reticulum stress. J. Neurosci. Res. 92, 1122–1133. doi: 10.1002/jnr.23389
Kawasaki, Y., Zhang, L., Cheng, J. K., and Ji, R. R. (2008). Cytokine mechanisms of central sensitization: distinct and overlapping role of interleukin-1beta, interleukin-6 and tumor necrosis factor-alpha in regulating synaptic and neuronal activity in the superficial spinal cord. J. Neurosci. 28, 5189–5194. doi: 10.1523/JNEUROSCI.3338-07.2008
Keinan, N., Pahima, H., Ben-Hail, D., and Shoshan-Barmatz, V. (2013). The role of calcium in VDAC1 oligomerization and mitochondria-mediated apoptosis. Biochim. Biophys. Acta 1833, 1745–1754. doi: 10.1016/j.bbamcr.2013.03.017
Kelly, B. L., and Ferreira, A. (2006). beta-Amyloid-induced dynamin 1 degradation is mediated by N-methyl-D-aspartate receptors in hippocampal neurons. J. Biol. Chem. 281, 28079–28089. doi: 10.1074/jbc.M605081200
Khan, M. T., and Joseph, S. K. (2010). Role of inositol trisphosphate receptors in autophagy in DT40 cells. J. Biol. Chem. 285, 16912–16920. doi: 10.1074/jbc.M110.114207
Kim, S. R., Kim, S. U., Oh, U., and Jin, B. K. (2006). Transient receptor potential vanilloid subtype 1 mediates microglial cell death in vivo and in vitro via Ca2+-mediated mitochondrial damage and cytochrome c release. J. Immunol. 177, 4322–4329. doi: 10.4049/jimmunol.177.7.4322
Kim, H. J., Soyombo, A. A., Tjon-Kon-Sang, S., So, I., and Muallem, S. (2009). The Ca(2+) channel TRPML3 regulates membrane trafficking and autophagy. Traffic 10, 1157–1167. doi: 10.1111/j.1600-0854.2009.00924.x
Kinjo, T., Ashida, Y., Higashi, H., Sugimura, S., Washida, M., Niihara, H., et al. (2018). Alleviation by GABA(B) receptors of neurotoxicity mediated by mitochondrial permeability transition pore in cultured murine cortical neurons exposed to N-Methyl-D-aspartate. Neurochem. Res. 43, 79–88. doi: 10.1007/s11064-017-2311-z
Klegeris, A., Choi, H. B., McLarnon, J. G., and McGeer, P. L. (2007). Functional ryanodine receptors are expressed by human microglia and THP-1 cells: their possible involvement in modulation of neurotoxicity. J. Neurosci. Res. 85, 2207–2215. doi: 10.1002/jnr.21361
Komita, M., Jin, H., and Aoe, T. (2013). The effect of endoplasmic reticulum stress on neurotoxicity caused by inhaled anesthetics. Anesth. Analg. 117, 1197–1204. doi: 10.1213/ANE.0b013e3182a74773
Komuro, H., and Rakic, P. (1992). Selective role of N-type calcium channels in neuronal migration. Science 257, 806–809. doi: 10.1126/science.1323145
Konno, M., Shirakawa, H., Iida, S., Sakimoto, S., Matsutani, I., Miyake, T., et al. (2012). Stimulation of transient receptor potential vanilloid 4 channel suppresses abnormal activation of microglia induced by lipopolysaccharide. Glia 60, 761–770. doi: 10.1002/glia.22306
Kouroku, Y., Fujita, E., Tanida, I., Ueno, T., Isoai, A., Kumagai, H., et al. (2007). ER stress (PERK/eIF2alpha phosphorylation) mediates the polyglutamine-induced LC3 conversion, an essential step for autophagy formation. Cell Death Differ. 14, 230–239. doi: 10.1038/sj.cdd.4401984
Kraft, R., Grimm, C., Grosse, K., Hoffmann, A., Sauerbruch, S., Kettenmann, H., et al. (2004). Hydrogen peroxide and ADP-ribose induce TRPM2-mediated calcium influx and cation currents in microglia. Am. J. Physiol. Cell Physiol. 286, C129–137. doi: 10.1152/ajpcell.00331.2003
Kraft, A., Jubal, E. R., von Laer, R., Döring, C., Rocha, A., Grebbin, M., et al. (2017). Astrocytic calcium waves signal brain injury to neural stem and progenitor cells. Stem Cell Rep. 8, 701–714. doi: 10.1016/j.stemcr.2017.01.009
Lashuel, H. A., Hartley, D. M., Petre, B. M., Wall, J. S., Simon, M. N., Walz, T., et al. (2003). Mixtures of wild-type and a pathogenic (E22G) form of Abeta40 in vitro accumulate protofibrils, including amyloid pores. J. Mol. Biol. 332, 795–808. doi: 10.1016/s0022-2836(03)00927-6
Lashuel, H. A., Hartley, D., Petre, B. M., Walz, T., and Lansbury, P. T.,Jr. (2002). Neurodegenerative disease: amyloid pores from pathogenic mutations. Nature 418:291. doi: 10.1038/418291a
Lawlor, B., Segurado, R., Kennelly, S., Olde Rikkert, M. G. M., Howard, R., Pasquier, F., et al. (2018). Nilvadipine in mild to moderate Alzheimer disease: a randomised controlled trial. PLoS Med. 15:e1002660. doi: 10.1371/journal.pmed.1002660
Lee, Y. J., Choi, S. Y., and Yang, J. H. (2016). AMP-activated protein kinase is involved in perfluorohexanesulfonate-induced apoptosis of neuronal cells. Chemosphere 149, 1–7. doi: 10.1016/j.chemosphere.2016.01.073
Lee, J. H., McBrayer, M. K., Wolfe, D. M., Haslett, L. J., Kumar, A., Sato, Y., et al. (2015). Presenilin 1 maintains lysosomal Ca(2+) homeostasis via TRPML1 by regulating vATPase-mediated lysosome acidification. Cell Rep. 12, 1430–1444. doi: 10.1016/j.celrep.2015.07.050
Lei, S. Z., Zhang, D., Abele, A. E., and Lipton, S. A. (1992). Blockade of NMDA receptor-mediated mobilization of intracellular Ca2+ prevents neurotoxicity. Brain Res. 598, 196–202. doi: 10.1016/0006-8993(92)90183-a
Leissring, M. A., Murphy, M. P., Mead, T. R., Akbari, Y., Sugarman, M. C., Jannatipour, M., et al. (2002). A physiologic signaling role for the gamma -secretase-derived intracellular fragment of APP. Proc. Natl. Acad. Sci. U S A 99, 4697–4702. doi: 10.1073/pnas.072033799
Li, X., and Jiang, L. H. (2018). Multiple molecular mechanisms form a positive feedback loop driving amyloid β42 peptide-induced neurotoxicity via activation of the TRPM2 channel in hippocampal neurons. Cell Death Dis. 9:195. doi: 10.1038/s41419-018-0270-1
Li, Y., and Jiao, J. (2020). Deficiency of TRPM2 leads to embryonic neurogenesis defects in hyperthermia. Sci. Adv. 6:eaay6350. doi: 10.1126/sciadv.aay6350
Li, R., Jin, Y., Li, Q., Sun, X., Zhu, H., and Cui, H. (2018). MiR-93–5p targeting PTEN regulates the NMDA-induced autophagy of retinal ganglion cells via AKT/mTOR pathway in glaucoma. Biomed. Pharmacother. 100, 1–7. doi: 10.1016/j.biopha.2018.01.044
Li, Y., Lin, X., Zhao, X., Xie, J., JunNan, W., Sun, T., et al. (2014). Ozone (O3) elicits neurotoxicity in spinal cord neurons (SCNs) by inducing ER Ca(2+) release and activating the CaMKII/MAPK signaling pathway. Toxicol. Appl. Pharmacol. 280, 493–501. doi: 10.1016/j.taap.2014.08.024
Li, L., Tsai, H. J., Li, L., and Wang, X. M. (2010). Icariin inhibits the increased inward calcium currents induced by amyloid-beta(25–35) peptide in CA1 pyramidal neurons of neonatal rat hippocampal slice. Am. J. Chin. Med. 38, 113–125. doi: 10.1142/S0192415X10007701
Li, T., Zhang, Y., Tian, J., Yang, L., and Wang, J. (2019). Ginkgo biloba pretreatment attenuates myocardial ischemia-reperfusion injury via mitoBK(Ca). Am. J. Chin. Med. 47, 1057–1073. doi: 10.1142/S0192415X1950054X
Lim, D., Dematteis, G., Tapella, L., Genazzani, A. A., Calì, T., Brini, M., et al. (2021a). Ca(2+) handling at the mitochondria-ER contact sites in neurodegeneration. Cell Calcium 98:102453. doi: 10.1016/j.ceca.2021.102453
Lim, D., Semyanov, A., Genazzani, A., and Verkhratsky, A. (2021b). Calcium signaling in neuroglia. Int. Rev. Cell Mol. Biol. 362, 1–53. doi: 10.1016/bs.ircmb.2021.01.003
Limke, T. L., Bearss, J. J., and Atchison, W. D. (2004). Acute exposure to methylmercury causes Ca2+ dysregulation and neuronal death in rat cerebellar granule cells through an M3 muscarinic receptor-linked pathway. Toxicol. Sci. 80, 60–68. doi: 10.1093/toxsci/kfh131
Lin, H., Bhatia, R., and Lal, R. (2001). Amyloid beta protein forms ion channels: implications for Alzheimer’s disease pathophysiology. FASEB J 15, 2433–2444. doi: 10.1096/fj.01-0377com
Liou, B., Peng, Y., Li, R., Inskeep, V., Zhang, W., Quinn, B., et al. (2016). Modulating ryanodine receptors with dantrolene attenuates neuronopathic phenotype in Gaucher disease mice. Hum. Mol. Genet. 25, 5126–5141. doi: 10.1093/hmg/ddw322
Liu, X., Du, H., Chen, D., Yuan, H., Chen, W., Jia, W., et al. (2019). Cyclophilin D deficiency protects against the development of mitochondrial ROS and cellular inflammation in aorta. Biochem. Biophys. Res. Commun. 508, 1202–1208. doi: 10.1016/j.bbrc.2018.12.064
Liu, H., Jia, X., Luo, Z., Guan, H., Jiang, H., Li, X., et al. (2012). Inhibition of store-operated Ca(2+) channels prevent ethanol-induced intracellular Ca(2+) increase and cell injury in a human hepatoma cell line. Toxicol. Lett 208, 254–261. doi: 10.1016/j.toxlet.2011.11.007
Liu, T., Jiang, C. Y., Fujita, T., Luo, S. W., and Kumamoto, E. (2013). Enhancement by interleukin-1β of AMPA and NMDA receptor-mediated currents in adult rat spinal superficial dorsal horn neurons. Mol. Pain 9:16. doi: 10.1186/1744-8069-9-16
Liu, X., Song, S., Wang, Q., Yuan, T., and He, J. (2016). A mutation in β-amyloid precursor protein renders SH-SY5Y cells vulnerable to isoflurane toxicity: the role of inositol 1,4,5-trisphosphate receptors. Mol. Med. Rep. 14, 5435–5442. doi: 10.3892/mmr.2016.5930
Liu, S. B., and Zhao, M. G. (2013). Neuroprotective effect of estrogen: role of nonsynaptic NR2B-containing NMDA receptors. Brain Res. Bull. 93, 27–31. doi: 10.1016/j.brainresbull.2012.10.004
Liu, S. J., and Zukin, R. S. (2007). Ca2+-permeable AMPA receptors in synaptic plasticity and neuronal death. Trends Neurosci. 30, 126–134. doi: 10.1016/j.tins.2007.01.006
Louhivuori, L. M., Jansson, L., Turunen, P. M., Jäntti, M. H., Nordström, T., Louhivuori, V., et al. (2015). Transient receptor potential channels and their role in modulating radial glial-neuronal interaction: a signaling pathway involving mGluR5. Stem Cells Dev. 24, 701–713. doi: 10.1089/scd.2014.0209
Louhivuori, L. M., Louhivuori, V., Wigren, H. K., Hakala, E., Jansson, L. C., Nordström, T., et al. (2013). Role of low voltage activated calcium channels in neuritogenesis and active migration of embryonic neural progenitor cells. Stem Cells Dev. 22, 1206–1219. doi: 10.1089/scd.2012.0234
Lu, C. W., Lin, T. Y., Chiu, K. M., Lee, M. Y., Huang, J. H., and Wang, S. J. (2020a). Silymarin inhibits glutamate release and prevents against kainic acid-induced excitotoxic injury in rats. Biomedicines 8:486. doi: 10.3390/biomedicines8110486
Lu, C. W., Lin, T. Y., Yang, H. C., Hung, C. F., Weng, J. R., Chang, C., et al. (2020b). [1–(4-chloro-3-nitrobenzenesulfonyl)-1H-indol-3-yl]-methanol, an indole-3-carbinol derivative, inhibits glutamate release in rat cerebrocortical nerve terminals by suppressing the P/Q-type Ca(2+) channels and Ca(2+)/calmodulin/protein kinase a pathway. Neurochem. Int. 140:104845. doi: 10.1016/j.neuint.2020.104845
Lu, C. W., Lin, T. Y., Wang, S. J., and Huang, S. K. (2019). Asiatic acid, an active substance of Centella asiatica, presynaptically depresses glutamate release in the rat hippocampus. Eur. J. Pharmacol. 865:172781. doi: 10.1016/j.ejphar.2019.172781
Ma, J., Brewer, H. B.Jr., and Potter, H. (1996). Alzheimer A beta neurotoxicity: promotion by antichymotrypsin, ApoE4; inhibition by A beta-related peptides. Neurobiol. Aging 17, 773–780. doi: 10.1016/0197-4580(96)00112-1
Ma, F., Zhang, L., and Westlund, K. N. (2009). Reactive oxygen species mediate TNFR1 increase after TRPV1 activation in mouse DRG neurons. Mol. Pain 5:31. doi: 10.1186/1744-8069-5-31
Ma, S. H., Zhuang, Q. X., Shen, W. X., Peng, Y. P., and Q25u, Y. H. (2015). Interleukin-6 reduces NMDAR-mediated cytosolic Ca2 overload and neuronal death via JAK/CaN signaling. Cell Calcium 58, 286–295. doi: 10.1016/j.ceca.2015.06.006
MacManus, A., Ramsden, M., Murray, M., Henderson, Z., Pearson, H. A., and Campbell, V. A. (2000). Enhancement of (45)Ca(2+) influx and voltage-dependent Ca(2+) channel activity by beta-amyloid-(1–40) in rat cortical synaptosomes and cultured cortical neurons. Modulation by the proinflammatory cytokine interleukin-1beta. J. Biol. Chem. 275, 4713–4718. doi: 10.1074/jbc.275.7.4713
Marcantoni, A., Cerullo, M. S., Buxeda, P., Tomagra, G., Giustetto, M., Chiantia, G., et al. (2020). Amyloid Beta42 oligomers up-regulate the excitatory synapses by potentiating presynaptic release while impairing postsynaptic NMDA receptors. J. Physiol. 598, 2183–2197. doi: 10.1113/JP279345
Marin, R., Ramírez, C. M., González, M., González-Muñoz, E., Zorzano, A., Camps, M., et al. (2007). Voltage-dependent anion channel (VDAC) participates in amyloid beta-induced toxicity and interacts with plasma membrane estrogen receptor alpha in septal and hippocampal neurons. Mol. Membr. Biol. 24, 148–160. doi: 10.1080/09687860601055559
Marques, M. A., and Crutcher, K. A. (2003). Apolipoprotein E-related neurotoxicity as a therapeutic target for Alzheimer’s disease. J. Mol. Neurosci. 20, 327–337. doi: 10.1385/JMN:20:3:327
Marschallinger, J., Sah, A., Schmuckermair, C., Unger, M., Rotheneichner, P., Kharitonova, M., et al. (2015). The L-type calcium channel Cav1.3 is required for proper hippocampal neurogenesis and cognitive functions. Cell Calcium 58, 606–616. doi: 10.1016/j.ceca.2015.09.007
Matsubara, M., Tamura, T., Ohmori, K., and Hasegawa, K. (2005). Histamine H1 receptor antagonist blocks histamine-induced proinflammatory cytokine production through inhibition of Ca2+-dependent protein kinase C, Raf/MEK/ERK and IKK/I kappa B/NF-kappa B signal cascades. Biochem. Pharmacol. 69, 433–449. doi: 10.1016/j.bcp.2004.10.006
Mattson, M. P., and Furukawa, K. (1997). Anti-apoptotic actions of cycloheximide: blockade of programmed cell death or induction of programmed cell life? Apoptosis 2, 257–264. doi: 10.1023/a:1026433019210
McBrayer, M., and Nixon, R. A. (2013). Lysosome and calcium dysregulation in Alzheimer’s disease: partners in crime. Biochem. Soc. Trans. 41, 1495–1502. doi: 10.1042/BST20130201
McDaid, J., Mustaly-Kalimi, S., and Stutzmann, G. E. (2020). Ca(2+) dyshomeostasis disrupts neuronal and synaptic function in Alzheimer’s disease. Cells 9:2655. doi: 10.3390/cells9122655
McLarnon, J. G., Franciosi, S., Wang, X., Bae, J. H., Choi, H. B., and Kim, S. U. (2001). Acute actions of tumor necrosis factor-alpha on intracellular Ca(2+) and K(+) currents in human microglia. Neuroscience 104, 1175–1184. doi: 10.1016/s0306-4522(01)00119-1
Mendez-David, I., Guilloux, J. P., Papp, M., Tritschler, L., Mocaer, E., Gardier, A.M., et al. (2017). S 47445 produces antidepressant- and anxiolytic-like effects through neurogenesis dependent and independent mechanisms. Front. Pharmacol. 8:462. doi: 10.3389/fphar.2017.00462
Meng, Y., Li, W. Z., Shi, Y. W., Zhou, B. F., Ma, R., and Li, W. P. (2016). Danshensu protects against ischemia/reperfusion injury and inhibits the apoptosis of H9c2 cells by reducing the calcium overload through the p-JNK-NF-κB-TRPC6 pathway. Int. J. Mol. Med. 37, 258–266. doi: 10.3892/ijmm.2015.2419
Miao, Y., Dong, L. D., Chen, J., Hu, X. C., Yang, X. L., and Wang, Z. (2012). Involvement of calpain/p35–p25/Cdk5/NMDAR signaling pathway in glutamate-induced neurotoxicity in cultured rat retinal neurons. PLoS One 7:e42318. doi: 10.1371/journal.pone.0042318
Michiels, C. F., Fransen, P., De Munck, D. G., De Meyer, G. R., and Martinet, W. (2015). Defective autophagy in vascular smooth muscle cells alters contractility and Ca2 homeostasis in mice. Am. J. Physiol. Heart Circ. Physiol. 308, H557–567. doi: 10.1152/ajpheart.00659.2014
Miyake, T., Shirakawa, H., Kusano, A., Sakimoto, S., Konno, M., Nakagawa, T., et al. (2014). TRPM2 contributes to LPS/IFNγ-induced production of nitric oxide via the p38/JNK pathway in microglia. Biochem. Biophys. Res. Commun. 444, 212–217. doi: 10.1016/j.bbrc.2014.01.022
Miyanohara, J., Kakae, M., Nagayasu, K., Nakagawa, T., Mori, Y., Arai, K., et al. (2018). TRPM2 channel aggravates CNS inflammation and cognitive impairment via activation of microglia in chronic cerebral hypoperfusion. J. Neurosci. 38, 3520–3533. doi: 10.1523/JNEUROSCI.2451-17.2018
Morais Cardoso, S., Swerdlow, R. H., and Oliveira, C. R. (2002). Induction of cytochrome c-mediated apoptosis by amyloid beta 25–35 requires functional mitochondria. Brain Res. 931, 117–125. doi: 10.1016/s0006-8993(02)02256-4
Morelli, M. B., Amantini, C., Liberati, S., Santoni, M., and Nabissi, M. (2013). TRP channels: new potential therapeutic approaches in CNS neuropathies. CNS Neurol. Disord. Drug Targets 12, 274–293. doi: 10.2174/18715273113129990056
Moreno, J.A., Halliday, M., Molloy, C., Radford, H., Verity, N., Axten, J. M., et al. (2013). Oral treatment targeting the unfolded protein response prevents neurodegeneration and clinical disease in prion-infected mice. Sci. Transl. Med. 5:206ra138. doi: 10.1126/scitranslmed.3006767
Murugan, M., Sivakumar, V., Lu, J., Ling, E. A., and Kaur, C. (2011). Expression of N-methyl D-aspartate receptor subunits in amoeboid microglia mediates production of nitric oxide via NF-κB signaling pathway and oligodendrocyte cell death in hypoxic postnatal rats. Glia 59, 521–539. doi: 10.1002/glia.21121
Muth-Köhne, E., Pachernegg, S., Karus, M., Faissner, A., and Hollmann, M. (2010). Expression of NMDA receptors and Ca2+-impermeable AMPA receptors requires neuronal differentiation and allows discrimination between two different types of neural stem cells. Cell Physiol. Biochem. 26, 935–946. doi: 10.1159/000324002
Nagakannan, P., Islam, M. I., Karimi-Abdolrezaee, S., and Eftekharpour, E. (2019). Inhibition of VDAC1 protects against glutamate-induced oxytosis and mitochondrial fragmentation in hippocampal HT22 cells. Cell Mol. Neurobiol. 39, 73–85. doi: 10.1007/s10571-018-0634-1
Nakamura, S., Shigeyama, S., Minami, S., Shima, T., Akayama, S., Matsuda, T., et al. (2020). LC3 lipidation is essential for TFEB activation during the lysosomal damage response to kidney injury. Nat. Cell Biol. 22, 1252–1263. doi: 10.1038/s41556-020-00583-9
Namba, T., Dóczi, J., Pinson, A., Xing, L., Kalebic, N., Wilsch-Bräuninger, M., et al. (2020). Human-specific ARHGAP11B acts in mitochondria to expand neocortical progenitors by glutaminolysis. Neuron 105, 867–881.e9. doi: 10.1016/j.neuron.2019.11.027
Nasiri-Ansari, N., Nikolopoulou, C., Papoutsi, K., Kyrou, I., Mantzoros, C. S., Kyriakopoulos, G., et al. (2021). Empagliflozin attenuates non-alcoholic fatty liver disease (NAFLD) in high fat diet fed ApoE((−/−)) mice by activating autophagy and reducing ER stress and apoptosis. Int. J. Mol. Sci. 22:818. doi: 10.3390/ijms22020818
Neumann, J., Sauerzweig, S., Rönicke, R., Gunzer, F., Dinkel, K., Ullrich, O., et al. (2008). Microglia cells protect neurons by direct engulfment of invading neutrophil granulocytes: a new mechanism of CNS immune privilege. J. Neurosci. 28, 5965–5975. doi: 10.1523/JNEUROSCI.0060-08.2008
Nijholt, D. A., de Graaf, T. R., van Haastert, E. S., Oliveira, A. O., Berkers, C. R., Zwart, R., et al. (2011). Endoplasmic reticulum stress activates autophagy but not the proteasome in neuronal cells: implications for Alzheimer’s disease. Cell Death Differ. 18, 1071–1081. doi: 10.1038/cdd.2010.176
Nishitoh, H., Kadowaki, H., Takeda, K., and Ichijo, H. (2009). ER quality control, ER stress-induced apoptosis and neurodegenerative diseases. Protein Misfolding Disord. Trip ER 94–102. doi: 10.2174/978160805013010901010094
Nursalim, Y. (2016). Preliminary Evidence for NMDAR Contribution Towards Autophagy and Membrane Potential in Leukaemic Megakaryoblasts. Masters Thesis. University of Auckland.
Ogoshi, F., Yin, H. Z., Kuppumbatti, Y., Song, B., Amindari, S., and Weiss, J. H. (2005). Tumor necrosis-factor-alpha (TNF-alpha) induces rapid insertion of Ca2+-permeable alpha-amino-3-hydroxyl-5-methyl-4-isoxazole-propionate (AMPA)/kainate (Ca-A/K) channels in a subset of hippocampal pyramidal neurons. Exp. Neurol. 193, 384–393. doi: 10.1016/j.expneurol.2004.12.026
Onyenwoke, R. U., Sexton, J. Z., Yan, F., Díaz, M. C., Forsberg, L. J., Major, M. B., et al. (2015). The mucolipidosis IV Ca2+ channel TRPML1 (MCOLN1) is regulated by the TOR kinase. Biochem. J. 470, 331–342. doi: 10.1042/BJ20150219
Orellana, D. I., Quintanilla, R. A., Gonzalez-Billault, C., and Maccioni, R. B. (2005). Role of the JAKs/STATs pathway in the intracellular calcium changes induced by interleukin-6 in hippocampal neurons. Neurotox. Res. 8, 295–304. doi: 10.1007/BF03033983
Oseki, K. T., Monteforte, P. T., Pereira, G. J., Hirata, H., Ureshino, R. P., Bincoletto, C., et al. (2014). Apoptosis induced by Aβ25–35 peptide is Ca(2+) -IP3 signaling-dependent in murine astrocytes. Eur. J. Neurosci. 40, 2471–2478. doi: 10.1111/ejn.12599
Ostapchenko, V. G., Chen, M., Guzman, M. S., Xie, Y. F., Lavine, N., Fan, J., et al. (2015). The transient receptor potential melastatin 2 (TRPM2) channel contributes to β-Amyloid oligomer-related neurotoxicity and memory impairment. J. Neurosci. 35, 15157–15169. doi: 10.1523/JNEUROSCI.4081-14.2015
Pahl, H. L., and Baeuerle, P. A. (1996). Activation of NF-kappa B by ER stress requires both Ca2+ and reactive oxygen intermediates as messengers. FEBS Lett 392, 129–136. doi: 10.1016/0014-5793(96)00800-9
Pahrudin Arrozi, A., Shukri, S. N. S., Wan Ngah, W. Z., Mohd Yusof, Y. A., Ahmad Damanhuri, M. H., Jaafar, F., et al. (2020). Comparative effects of alpha- and gamma-tocopherol on mitochondrial functions in Alzheimer’s disease in vitro model. Sci. Rep. 10:8962. doi: 10.1038/s41598-020-65570-4
Park, E. S., Kim, S. R., and Jin, B. K. (2012). Transient receptor potential vanilloid subtype 1 contributes to mesencephalic dopaminergic neuronal survival by inhibiting microglia-originated oxidative stress. Brain Res. Bull. 89, 92–96. doi: 10.1016/j.brainresbull.2012.07.001
Park, K. M., Yule, D. I., and Bowers, W. J. (2008). Tumor necrosis factor-alpha potentiates intraneuronal Ca2+ signaling via regulation of the inositol 1,4,5-trisphosphate receptor. J. Biol. Chem. 283, 33069–33079. doi: 10.1074/jbc.M802209200
Park, K. M., Yule, D. I., and Bowers, W. J. (2010). Impaired TNF-alpha control of IP3R-mediated Ca2+ release in Alzheimer’s disease mouse neurons. Cell Signal 22, 519–526. doi: 10.1016/j.cellsig.2009.11.006
Pedrozo, Z., Torrealba, N., Fernández, C., Gatica, D., Toro, B., Quiroga, C., et al. (2013). Cardiomyocyte ryanodine receptor degradation by chaperone-mediated autophagy. Cardiovasc. Res. 98, 277–285. doi: 10.1093/cvr/cvt029
Peng, Y., Cheung, K. H., Liang, G., Inan, S., Vais, H., Joseph, D., et al. (2011). General anesthetics influence autophagy and neurodegeneration through actions on the inositol 1,4,5-trisphosphate receptor calcium channel. Alzheimer’s Demen. 7:S573. doi: 10.1016/j.jalz.2011.05.1618
Piacentini, R., Gangitano, C., Ceccariglia, S., Del Fà, A., Azzena, G. B., Michetti, F., et al. (2008a). Dysregulation of intracellular calcium homeostasis is responsible for neuronal death in an experimental model of selective hippocampal degeneration induced by trimethyltin. J. Neurochem. 105, 2109–2121. doi: 10.1111/j.1471-4159.2008.05297.x
Piacentini, R., Ripoli, C., Leone, L., Misiti, F., Clementi, M. E., D’Ascenzo, M., et al. (2008b). Role of methionine 35 in the intracellular Ca2+ homeostasis dysregulation and Ca2+-dependent apoptosis induced by amyloid beta-peptide in human neuroblastoma IMR32 cells. J. Neurochem. 107, 1070–1082. doi: 10.1111/j.1471-4159.2008.05680.x
Piacentini, R., Ripoli, C., Mezzogori, D., Azzena, G. B., and Grassi, C. (2008c). Extremely low-frequency electromagnetic fields promote in vitro neurogenesis via upregulation of Ca(v)1-channel activity. J. Cell Physiol. 215, 129–139. doi: 10.1002/jcp.21293
Pickford, F., Masliah, E., Britschgi, M., Lucin, K., Narasimhan, R., Jaeger, P. A., et al. (2008). The autophagy-related protein beclin 1 shows reduced expression in early Alzheimer disease and regulates amyloid beta accumulation in mice. J. Clin. Invest. 118, 2190–2199. doi: 10.1172/JCI33585
Pierrot, N., Ghisdal, P., Caumont, A. S., and Octave, J. N. (2004). Intraneuronal amyloid-beta1–42 production triggered by sustained increase of cytosolic calcium concentration induces neuronal death. J. Neurochem. 88, 1140–1150. doi: 10.1046/j.1471-4159.2003.02227.x
Pitt, D., Werner, P., and Raine, C. S. (2000). Glutamate excitotoxicity in a model of multiple sclerosis. Nat. Med. 6, 67–70. doi: 10.1038/71555
Pollock, J., McFarlane, S. M., Connell, M. C., Zehavi, U., Vandenabeele, P., MacEwan, D. J., et al. (2002). TNF-alpha receptors simultaneously activate Ca2+ mobilisation and stress kinases in cultured sensory neurones. Neuropharmacology 42, 93–106. doi: 10.1016/s0028-3908(01)00163-0
Qiao, H., Li, Y., Xu, Z., Li, W., Fu, Z., Wang, Y., et al. (2017). Propofol affects neurodegeneration and neurogenesis by regulation of autophagy via effects on intracellular calcium homeostasis. Anesthesiology 127, 490–501. doi: 10.1097/ALN.0000000000001730
Qin, L., Wang, Z., Tao, L., and Wang, Y. (2010). ER stress negatively regulates AKT/TSC/mTOR pathway to enhance autophagy. Autophagy 6, 239–247. doi: 10.4161/auto.6.2.11062
Qu, M., Zhou, Z., Chen, C., Li, M., Pei, L., Yang, J., et al. (2012). Inhibition of mitochondrial permeability transition pore opening is involved in the protective effects of mortalin overexpression against beta-amyloid-induced apoptosis in SH-SY5Y cells. Neurosci. Res. 72, 94–102. doi: 10.1016/j.neures.2011.09.009
Raghunatha, P., Vosoughi, A., Kauppinen, T. M., and Jackson, M. F. (2020). Microglial NMDA receptors drive pro-inflammatory responses via PARP-1/TRMP2 signaling. Glia 68, 1421–1434. doi: 10.1002/glia.23790
Rainey-Smith, S. R., Andersson, D. A., Williams, R. J., and Rattray, M. (2010). Tumour necrosis factor alpha induces rapid reduction in AMPA receptor-mediated calcium entry in motor neurones by increasing cell surface expression of the GluR2 subunit: relevance to neurodegeneration. J. Neurochem. 113, 692–703. doi: 10.1111/j.1471-4159.2010.06634.x
Rash, B. G., Ackman, J. B., and Rakic, P. (2016). Bidirectional radial Ca(2+) activity regulates neurogenesis and migration during early cortical column formation. Sci. Adv. 2:e1501733. doi: 10.1126/sciadv.1501733
Resende, R. R., da Costa, J. L., Kihara, A. H., Adhikari, A., and Lorençon, E. (2010). Intracellular Ca2+ regulation during neuronal differentiation of murine embryonal carcinoma and mesenchymal stem cells. Stem Cells Dev. 19, 379–394. doi: 10.1089/scd.2008.0289
Rijpma, A., Jansen, D., Arnoldussen, I. A., Fang, X. T., Wiesmann, M., Mutsaers, M. P., et al. (2013). Sex differences in presynaptic density and neurogenesis in middle-aged ApoE4 and ApoE knockout mice. J. Neurodegener. Dis. 2013:531326. doi: 10.1155/2013/531326
Rodriguez, G. A., Tai, L. M., LaDu, M. J., and Rebeck, G. W. (2014). Human APOE4 increases microglia reactivity at Aβ plaques in a mouse model of Aβ deposition. J. Neuroinflammation 11:111. doi: 10.1186/1742-2094-11-111
Rostovtseva, T. K., Antonsson, B., Suzuki, M., Youle, R. J., Colombini, M., and Bezrukov, S. M. (2004). Bid, but not bax, regulates VDAC channels. J. Biol. Chem. 279, 13575–13583. doi: 10.1074/jbc.M310593200
Sade, Y., Toker, L., Kara, N.Z., Einat, H., Rapoport, S., Moechars, D., et al. (2016). IP3 accumulation and/or inositol depletion: two downstream lithium’s effects that may mediate its behavioral and cellular changes. Transl. Psychiatry 6:e968. doi: 10.1038/tp.2016.217
Sakamoto, K., Kawakami, T., Shimada, M., Yamaguchi, A., Kuwagata, M., Saito, M., et al. (2009). Histological protection by cilnidipine, a dual L/N-type Ca(2+) channel blocker, against neurotoxicity induced by ischemia-reperfusion in rat retina. Exp. Eye Res. 88, 974–982. doi: 10.1016/j.exer.2008.12.011
Sama, M. A., Mathis, D. M., Furman, J. L., Abdul, H. M., Artiushin, I. A., Kraner, S. D., et al. (2008). Interleukin-1beta-dependent signaling between astrocytes and neurons depends critically on astrocytic calcineurin/NFAT activity. J. Biol. Chem. 283, 21953–21964. doi: 10.1074/jbc.M800148200
Sama, D. M., Mohmmad Abdul, H., Furman, J. L., Artiushin, I. A., Szymkowski, D. E., Scheff, S. W., et al. (2012). Inhibition of soluble tumor necrosis factor ameliorates synaptic alterations and Ca2+ dysregulation in aged rats. PLoS One 7:e38170. doi: 10.1371/journal.pone.0038170
Sama, D. M., and Norris, C. M. (2013). Calcium dysregulation and neuroinflammation: discrete and integrated mechanisms for age-related synaptic dysfunction. Ageing Res. Rev. 12, 982–995. doi: 10.1016/j.arr.2013.05.008
Sanchez, A. B., Medders, K. E., Maung, R., Sánchez-Pavón, P., Ojeda-Juárez, D., and Kaul, M. (2016). CXCL12-induced neurotoxicity critically depends on NMDA receptor-gated and L-type Ca(2+) channels upstream of p38 MAPK. J. Neuroinflammation 13:252. doi: 10.1186/s12974-016-0724-2
Santoro, M., Piacentini, R., Perna, A., Pisano, E., and Silvestri, G. (2020). Resveratrol corrects aberrant splicing of RYR1 pre-mRNA and Ca 2+ signal in myotonic dystrophy type 1 myotubes. Neural Regen. Res. 15, 1757–1766. doi: 10.4103/1673-5374.276336
Sappington, R. M., and Calkins, D. J. (2008). Contribution of TRPV1 to microglia-derived IL-6 and NFkappaB translocation with elevated hydrostatic pressure. Invest. Ophthalmol. Vis. Sci. 49, 3004–3017. doi: 10.1167/iovs.07-1355
Sarkar, S., Floto, R. A., Berger, Z., Imarisio, S., Cordenier, A., Pasco, M., et al. (2005). Lithium induces autophagy by inhibiting inositol monophosphatase. J. Cell Biol. 170, 1101–1111. doi: 10.1083/jcb.200504035
Schmukler, E., Solomon, S., Simonovitch, S., Goldshmit, Y., Wolfson, E., Michaelson, D. M., et al. (2020). Altered mitochondrial dynamics and function in APOE4-expressing astrocytes. Cell Death Dis. 11:578. doi: 10.1038/s41419-020-02776-4
Selvaraj, S., Sun, Y., Sukumaran, P., and Singh, B. B. (2016). Resveratrol activates autophagic cell death in prostate cancer cells via downregulation of STIM1 and the mTOR pathway. Mol. Carcinog. 55, 818–831. doi: 10.1002/mc.22324
Seo, H., and Lee, K. (2016). Epac2 contributes to PACAP-induced astrocytic differentiation through calcium ion influx in neural precursor cells. BMB Rep. 49, 128–133. doi: 10.5483/bmbrep.2016.49.2.202
Shaikh, S., Troncoso, R., Criollo, A., Bravo-Sagua, R., García, L., Morselli, E., et al. (2016). Regulation of cardiomyocyte autophagy by calcium. Am. J. Physiol. Endocrinol. Metab. 310, E587–E596. doi: 10.1152/ajpendo.00374.2015
Shehata, M., Matsumura, H., Okubo-Suzuki, R., Ohkawa, N., and Inokuchi, K. (2012). Neuronal stimulation induces autophagy in hippocampal neurons that is involved in AMPA receptor degradation after chemical long-term depression. J. Neurosci. 32, 10413–10422. doi: 10.1523/JNEUROSCI.4533-11.2012
Shi, M., Du, F., Liu, Y., Li, L., Cai, J., Zhang, G. F., et al. (2013). Glial cell-expressed mechanosensitive channel TRPV4 mediates infrasound-induced neuronal impairment. Acta Neuropathol. 126, 725–739. doi: 10.1007/s00401-013-1166-x
Shimizu, S., Narita, M., and Tsujimoto, Y. (1999). Bcl-2 family proteins regulate the release of apoptogenic cytochrome c by the mitochondrial channel VDAC. Nature 399, 483–487. doi: 10.1038/20959
Shirakawa, H., Yamaoka, T., Sanpei, K., Nakagawa, T., and Kaneko, S. (2007). TRPV1 mediates vanilloids and low pH-induced neurotoxicity in rat cortical cultures. Neurosci. Res. 58, S207–S207. doi: 10.1016/j.neures.2007.06.946
Shoshan-Barmatz, V., Nahon-Crystal, E., Shteinfer-Kuzmine, A., and Gupta, R. (2018). VDAC1, mitochondrial dysfunction and Alzheimer’s disease. Pharmacol. Res. 131, 87–101. doi: 10.1016/j.phrs.2018.03.010
Shtaya, A., Sadek, A. R., Zaben, M., Seifert, G., Pringle, A., Steinhäuser, C., et al. (2018). AMPA receptors and seizures mediate hippocampal radial glia-like stem cell proliferation. Glia 66, 2397–2413. doi: 10.1002/glia.23479
Simões, A.P., Duarte, J.A., Agasse, F., Canas, P.M., Tomé, A.R., Agostinho, P., et al. (2012). Blockade of adenosine A2A receptors prevents interleukin-1β-induced exacerbation of neuronal toxicity through a p38 mitogen-activated protein kinase pathway. J. Neuroinflammation 9:204. doi: 10.1177/00031348211048845
Simonovitch, S., Schmukler, E., Bespalko, A., Iram, T., Frenkel, D., Holtzman, D. M., et al. (2016). Impaired autophagy in APOE4 astrocytes. J. Alzheimers Dis. 51, 915–927. doi: 10.3233/JAD-151101
Smilansky, A., Dangoor, L., Nakdimon, I., Ben-Hail, D., Mizrachi, D., and Shoshan-Barmatz, V. (2015). The voltage-dependent anion channel 1 mediates amyloid β toxicity and represents a potential target for alzheimer disease therapy. J. Biol. Chem. 290, 30670–30683. doi: 10.1074/jbc.M115.691493
Smith, T., Groom, A., Zhu, B., and Turski, L. (2000). Autoimmune encephalomyelitis ameliorated by AMPA antagonists. Nat. Med. 6, 62–66. doi: 10.1038/71548
Soboloff, J., and Berger, S. A. (2002). Sustained ER Ca2+ depletion suppresses protein synthesis and induces activation-enhanced cell death in mast cells. J. Biol. Chem. 277, 13812–13820. doi: 10.1074/jbc.M112129200
Somasundaram, A., Shum, A. K., McBride, H. J., Kessler, J. A., Feske, S., Miller, R. J., et al. (2014). Store-operated CRAC channels regulate gene expression and proliferation in neural progenitor cells. J. Neurosci. 34, 9107–9123. doi: 10.1523/JNEUROSCI.0263-14.2014
Song, S., Lee, H., Kam, T. I., Tai, M. L., Lee, J. Y., Noh, J. Y., et al. (2008). E2–25K/Hip-2 regulates caspase-12 in ER stress-mediated Abeta neurotoxicity. J. Cell Biol. 182, 675–684. doi: 10.1083/jcb.200711066
Song, Y., Li, D., Farrelly, O., Miles, L., Li, F., Kim, S. E., et al. (2019). The mechanosensitive ion channel piezo inhibits axon regeneration. Neuron 102, 373–389.e376. doi: 10.1016/j.neuron.2019.01.050
Staats, K. A., Humblet-Baron, S., Bento-Abreu, A., Scheveneels, W., Nikolaou, A., Deckers, K., et al. (2016). Genetic ablation of IP3 receptor 2 increases cytokines and decreases survival of SOD1G93A mice. Hum. Mol. Genet. 25, 3491–3499. doi: 10.1093/hmg/ddw190
Sukumaran, P., Schaar, A., Sun, Y., and Singh, B. B. (2016). Functional role of TRP channels in modulating ER stress and Autophagy. Cell Calcium 60, 123–132. doi: 10.1016/j.ceca.2016.02.012
Sun, G. B., Sun, H., Meng, X. B., Hu, J., Zhang, Q., Liu, B., et al. (2014). Aconitine-induced Ca2+ overload causes arrhythmia and triggers apoptosis through p38 MAPK signaling pathway in rats. Toxicol. Appl. Pharmacol. 279, 8–22. doi: 10.1016/j.taap.2014.05.005
Sun, Y., Chauhan, A., Sukumaran, P., Sharma, J., Singh, B. B., and Mishra, B. B. (2014). Inhibition of store-operated calcium entry in microglia by helminth factors: implications for immune suppression in neurocysticercosis. J. Neuroinflammation 11:210. doi: 10.1186/s12974-014-0210-7
Sun, Y., Vashisht, A. A., Tchieu, J., Wohlschlegel, J. A., and Dreier, L. (2012). Voltage-dependent anion channels (VDACs) recruit Parkin to defective mitochondria to promote mitochondrial autophagy. J. Biol. Chem. 287, 40652–40660. doi: 10.1074/jbc.M112.419721
Supnet, C., Noonan, C., Richard, K., Bradley, J., and Mayne, M. (2010). Up-regulation of the type 3 ryanodine receptor is neuroprotective in the TgCRND8 mouse model of Alzheimer’s disease. J. Neurochem. 112, 356–365. doi: 10.1111/j.1471-4159.2009.06487.x
Szalai, G., Krishnamurthy, R., and Hajnóczky, G. (1999). Apoptosis driven by IP(3)-linked mitochondrial calcium signals. EMBO J. 18, 6349–6361. doi: 10.1093/emboj/18.22.6349
Takeo, Y., Kurabayashi, N., Nguyen, M. D., and Sanada, K. (2016). The G protein-coupled receptor GPR157 regulates neuronal differentiation of radial glial progenitors through the Gq-IP3 pathway. Sci. Rep. 6:25180. doi: 10.1038/srep25180
Temme, S. J., Bell, R. Z., Fisher, G. L., and Murphy, G. G. (2016). Deletion of the mouse homolog of CACNA1C disrupts discrete forms of hippocampal-dependent memory and neurogenesis within the dentate gyrus. eNeuro 3:ENEURO.0118-16.2016. doi: 10.1523/ENEURO.0118-16.2016
Tharmalingam, S., Wu, C., and Hampson, D. R. (2016). The calcium-sensing receptor and integrins modulate cerebellar granule cell precursor differentiation and migration. Dev. Neurobiol. 76, 375–389. doi: 10.1002/dneu.22321
Thinnes, F. P. (2011). Apoptogenic interactions of plasmalemmal type-1 VDAC and Aβ peptides via GxxxG motifs induce Alzheimer’s disease - a basic model of apoptosis. Wien Med. Wochenschr. 161, 274–276. doi: 10.1007/s10354-011-0887-5
Thomas, M. P., and Morrisett, R. A. (2000). Dynamics of NMDAR-mediated neurotoxicity during chronic ethanol exposure and withdrawal. Neuropharmacology 39, 218–226. doi: 10.1016/s0028-3908(99)00107-0
Tolar, M., Keller, J. N., Chan, S., Mattson, M. P., Marques, M. A., and Crutcher, K. A. (1999). Truncated apolipoprotein E (ApoE) causes increased intracellular calcium and may mediate ApoE neurotoxicity. J. Neurosci. 19, 7100–7110. doi: 10.1523/JNEUROSCI.19-16-07100.1999
Tong, B. C., Wu, A. J., Li, M., and Cheung, K. H. (2018). Calcium signaling in Alzheimer’s disease and therapies. Biochim. Biophys. Acta Mol. Cell Res. 1865, 1745–1760. doi: 10.1016/j.bbamcr.2018.07.018
Toth, A. B., Shum, A. K., and Prakriya, M. (2016). Regulation of neurogenesis by calcium signaling. Cell Calcium 59, 124–134. doi: 10.1016/j.ceca.2016.02.011
Turovskaya, M. V., Turovsky, E. A., Zinchenko, V. P., Levin, S. G., and Godukhin, O. V. (2012). Interleukin-10 modulates [Ca2+]i response induced by repeated NMDA receptor activation with brief hypoxia through inhibition of InsP(3)-sensitive internal stores in hippocampal neurons. Neurosci. Lett. 516, 151–155. doi: 10.1016/j.neulet.2012.03.084
Ueda, K., Shinohara, S., Yagami, T., Asakura, K., and Kawasaki, K. (1997). Amyloid beta protein potentiates Ca2+ influx through L-type voltage-sensitive Ca2+ channels: a possible involvement of free radicals. J. Neurochem. 68, 265–271. doi: 10.1046/j.1471-4159.1997.68010265.x
Valladares, D., Utreras-Mendoza, Y., Campos, C., Morales, C., Diaz-Vegas, A., Contreras-Ferrat, A., et al. (2018). IP(3) receptor blockade restores autophagy and mitochondrial function in skeletal muscle fibers of dystrophic mice. Biochim. Biophys. Acta Mol. Basis. Dis. 1864, 3685–3695. doi: 10.1016/j.bbadis.2018.08.042
Vander Heiden, M. G., Li, X. X., Gottleib, E., Hill, R. B., Thompson, C. B., and Colombini, M. (2001). Bcl-xL promotes the open configuration of the voltage-dependent anion channel and metabolite passage through the outer mitochondrial membrane. J. Biol. Chem. 276, 19414–19419. doi: 10.1074/jbc.M101590200
Veinbergs, I., Everson, A., Sagara, Y., and Masliah, E. (2002). Neurotoxic effects of apolipoprotein E4 are mediated via dysregulation of calcium homeostasis. J. Neurosci. Res. 67, 379–387. doi: 10.1002/jnr.10138
Vergarajauregui, S., Connelly, P. S., Daniels, M. P., and Puertollano, R. (2008). Autophagic dysfunction in mucolipidosis type IV patients. Hum. Mol. Genet. 17, 2723–2737. doi: 10.1093/hmg/ddn174
Vervliet, T., Pintelon, I., Welkenhuyzen, K., Bootman, M. D., Bannai, H., Mikoshiba, K., et al. (2017). Basal ryanodine receptor activity suppresses autophagic flux. Biochem. Pharmacol. 132, 133–142. doi: 10.1016/j.bcp.2017.03.011
Vierling, C., Baumgartner, C. M., Bollerhey, M., Erhardt, W. D., Stampfl, A., and Vierling, W. (2014). The vasodilating effect of a Hintonia latiflora extract with antidiabetic action. Phytomedicine 21, 1582–1586. doi: 10.1016/j.phymed.2014.07.009
Villegas, S., Villarreal, F. J., and Dillmann, W. H. (2000). Leukemia inhibitory factor and Interleukin-6 downregulate sarcoplasmic reticulum Ca2+ ATPase (SERCA2) in cardiac myocytes. Basic Res. Cardiol. 95, 47–54. doi: 10.1007/s003950050007
Viviani, B., Bartesaghi, S., Gardoni, F., Vezzani, A., Behrens, M. M., Bartfai, T., et al. (2003). Interleukin-1beta enhances NMDA receptor-mediated intracellular calcium increase through activation of the Src family of kinases. J. Neurosci. 23, 8692–8700. doi: 10.1523/JNEUROSCI.23-25-08692.2003
Viviani, B., Gardoni, F., Bartesaghi, S., Corsini, E., Facchi, A., Galli, C. L., et al. (2006). Interleukin-1 beta released by gp120 drives neural death through tyrosine phosphorylation and trafficking of NMDA receptors. J. Biol. Chem. 281, 30212–30222. doi: 10.1074/jbc.M602156200
Vogl, C., Mochida, S., Wolff, C., Whalley, B. J., and Stephens, G. J. (2012). The synaptic vesicle glycoprotein 2A ligand levetiracetam inhibits presynaptic Ca2+ channels through an intracellular pathway. Mol. Pharmacol. 82, 199–208. doi: 10.1124/mol.111.076687
Wahlestedt, C., Golanov, E., Yamamoto, S., Yee, F., Ericson, H., Yoo, H., et al. (1993). Antisense oligodeoxynucleotides to NMDA-R1 receptor channel protect cortical neurons from excitotoxicity and reduce focal ischaemic infarctions. Nature 363, 260–263. doi: 10.1038/363260a0
Wallström, E., Diener, P., Ljungdahl, A., Khademi, M., Nilsson, C. G., and Olsson, T. (1996). Memantine abrogates neurological deficits, but not CNS inflammation, in Lewis rat experimental autoimmune encephalomyelitis. J. Neurol. Sci. 137, 89–96. doi: 10.3390/ijms22105335
Wang, W., Gao, Q., Yang, M., Zhang, X., Yu, L., Lawas, M., et al. (2015). Up-regulation of lysosomal TRPML1 channels is essential for lysosomal adaptation to nutrient starvation. Proc. Natl. Acad. Sci. U S A 112, E1373–1381. doi: 10.1073/pnas.1419669112
Wang, S. E., Ko, S. Y., Kim, Y. S., Jo, S., Lee, S. H., Jung, S. J., et al. (2018). Capsaicin upregulates HDAC2 via TRPV1 and impairs neuronal maturation in mice. Exp. Mol. Med. 50:e455. doi: 10.1038/emm.2017.289
Wang, D. M., Li, S. Q., Zhu, X. Y., Wang, Y., Wu, W. L., and Zhang, X. J. (2013). Protective effects of hesperidin against amyloid-β (Aβ) induced neurotoxicity through the voltage dependent anion channel 1 (VDAC1)-mediated mitochondrial apoptotic pathway in PC12 cells. Neurochem. Res. 38, 1034–1044. doi: 10.1007/s11064-013-1013-4
Wang, Z., Liu, S., Kakizaki, M., Hirose, Y., Ishikawa, Y., Funato, H., et al. (2014). Orexin/hypocretin activates mTOR complex 1 (mTORC1) via an Erk/Akt-independent and calcium-stimulated lysosome v-ATPase pathway. J. Biol. Chem. 289, 31950–31959. doi: 10.1074/jbc.M114.600015
Wang, P., and Wang, Z. Y. (2017). Metal ions influx is a double edged sword for the pathogenesis of Alzheimer’s disease. Ageing Res. Rev. 35, 265–290. doi: 10.1016/j.arr.2016.10.003
Wang, Q., Yang, L., and Wang, Y. (2015). Enhanced differentiation of neural stem cells to neurons and promotion of neurite outgrowth by oxygen-glucose deprivation. Int. J. Dev. Neurosci. 43, 50–57. doi: 10.1016/j.ijdevneu.2015.04.009
Wang, G., Zhang, J., Xu, C., Han, X., Gao, Y., and Chen, H. (2016). Inhibition of SOCs attenuates acute lung injury induced by severe acute pancreatitis in rats and PMVECs injury induced by lipopolysaccharide. Inflammation 39, 1049–1058. doi: 10.1007/s10753-016-0335-1
Wang, Z. J., Zhao, F., Wang, C. F., Zhang, X. M., Xiao, Y., Zhou, F., et al. (2019). Xestospongin C, a reversible IP3 receptor antagonist, alleviates the cognitive and pathological impairments in APP/PS1 mice of Alzheimer’s disease. J. Alzheimers Dis. 72, 1217–1231. doi: 10.3233/JAD-190796
Wang, X., and Zheng, W. (2019). Ca(2+) homeostasis dysregulation in Alzheimer’s disease: a focus on plasma membrane and cell organelles. FASEB J 33, 6697–6712. doi: 10.1096/fj.201801751R
Wei, H., Liang, G., Yang, H., Wang, Q., Hawkins, B., Madesh, M., et al. (2008). The common inhalational anesthetic isoflurane induces apoptosis via activation of inositol 1,4,5-trisphosphate receptors. Anesthesiology 108, 251–260. doi: 10.1097/01.anes.0000299435.59242.0e
Weissman, T. A., Riquelme, P. A., Ivic, L., Flint, A. C., and Kriegstein, A. R. (2004). Calcium waves propagate through radial glial cells and modulate proliferation in the developing neocortex. Neuron 43, 647–661. doi: 10.1016/j.neuron.2004.08.015
Weisthal, S., Keinan, N., Ben-Hail, D., Arif, T., and Shoshan-Barmatz, V. (2014). Ca(2+)-mediated regulation of VDAC1 expression levels is associated with cell death induction. Biochim. Biophys. Acta 1843, 2270–2281. doi: 10.1016/j.bbamcr.2014.03.021
Whitney, N. P., Peng, H., Erdmann, N. B., Tian, C., Monaghan, D. T., and Zheng, J. C. (2008). Calcium-permeable AMPA receptors containing Q/R-unedited GluR2 direct human neural progenitor cell differentiation to neurons. FASEB J. 22, 2888–2900. doi: 10.1096/fj.07-104661
Wigerblad, G., Huie, J. R., Yin, H. Z., Leinders, M., Pritchard, R. A., Koehrn, F. J., et al. (2017). Inflammation-induced GluA1 trafficking and membrane insertion of Ca(2+) permeable AMPA receptors in dorsal horn neurons is dependent on spinal tumor necrosis factor, PI3 kinase and protein kinase A. Exp. Neurol. 293, 144–158. doi: 10.1016/j.expneurol.2017.04.004
Williams, A., Sarkar, S., Cuddon, P., Ttofi, E. K., Saiki, S., Siddiqi, F. H., et al. (2008). Novel targets for Huntington’s disease in an mTOR-independent autophagy pathway. Nat. Chem. Biol. 4, 295–305. doi: 10.1038/nchembio.79
Wong, A., Grubb, D. R., Cooley, N., Luo, J., and Woodcock, E. A. (2013). Regulation of autophagy in cardiomyocytes by Ins(1,4,5)P(3) and IP(3)-receptors. J. Mol. Cell Cardiol. 54, 19–24. doi: 10.1016/j.yjmcc.2012.10.014
Wu, H. Y., Huang, C. H., Lin, Y. H., Wang, C. C., and Jan, T. R. (2018). Cannabidiol induced apoptosis in human monocytes through mitochondrial permeability transition pore-mediated ROS production. Free Radic. Biol. Med. 124, 311–318. doi: 10.1016/j.freeradbiomed.2018.06.023
Xia, Y., Ragan, R. E., Seah, E. E., Michaelis, M. L., and Michaelis, E. K. (1995). Developmental expression of N-methyl-D-aspartate (NMDA)-induced neurotoxicity, NMDA receptor function and the NMDAR1 and glutamate-binding protein subunits in cerebellar granule cells in primary cultures. Neurochem. Res. 20, 617–629. doi: 10.1007/BF01694545
Xu, H. X., Cui, S. M., Zhang, Y. M., and Ren, J. (2020). Mitochondrial Ca(2+) regulation in the etiology of heart failure: physiological and pathophysiological implications. Acta Pharmacol. Sin. 41, 1301–1309. doi: 10.1038/s41401-020-0476-5
Xu, D., and Peng, Y. (2017). Apolipoprotein E 4 triggers multiple pathway-mediated Ca2+ overload, causes CaMK II phosphorylation abnormity and aggravates oxidative stress caused cerebral cortical neuron damage. Eur. Rev. Med. Pharmacol. Sci. 21, 5717–5728. doi: 10.26355/eurrev_201712_14018
Xu, Y. Y., Wan, W. P., Zhao, S., and Ma, Z. G. (2020). L-type calcium channels are involved in iron-induced neurotoxicity in primary cultured ventral mesencephalon neurons of rats. Neurosci. Bull. 36, 165–173. doi: 10.1007/s12264-019-00424-2
Xue, Z., Guo, Y., and Fang, Y. (2016). Moderate activation of autophagy regulates the intracellular calcium ion concentration and mitochondrial membrane potential in beta-amyloid-treated PC12 cells. Neurosci. Lett. 618, 50–57. doi: 10.1016/j.neulet.2016.02.044
Yagami, T., Ueda, K., Asakura, K., Kuroda, T., Hata, S., Sakaeda, T., et al. (2002). Effects of endothelin B receptor agonists on amyloid beta protein (25–35)-induced neuronal cell death. Brain Res. 948, 72–81. doi: 10.1016/s0006-8993(02)02951-7
Yang, C. W., Borowitz, J. L., Gunasekar, P. G., and Isom, G. E. (1996). Cyanide-stimulated inositol 1,4,5-trisphosphate formation: an intracellular neurotoxic signaling cascade. J. Biochem. Toxicol. 11, 251–256. doi: 10.1002/(SICI)1522-7146(1996)11:5<251::AID-JBT6>3.0.CO;2-J
Yang, B., Li, J. J., Cao, J. J., Yang, C. B., Liu, J., Ji, Q. M., et al. (2013). Polydatin attenuated food allergy via store-operated calcium channels in mast cell. World J. Gastroenterol. 19, 3980–3989. doi: 10.3748/wjg.v19.i25.3980
Yang, Z. Y., Liu, J., and Chu, H. C. (2020). Effect of NMDAR-NMNAT1/2 pathway on neuronal cell damage and cognitive impairment of sevoflurane-induced aged rats. Neurol. Res. 42, 108–117. doi: 10.1080/01616412.2019.1710393
Yang, N. N., Shi, H., Yu, G., Wang, C. M., Zhu, C., Yang, Y., et al. (2016). Osthole inhibits histamine-dependent itch via modulating TRPV1 activity. Sci. Rep. 6:25657. doi: 10.1038/srep25657
Yang, J., Wang, R., Cheng, X., Qu, H., Qi, J., Li, D., et al. (2020). The vascular dilatation induced by Hydroxysafflor yellow A (HSYA) on rat mesenteric artery through TRPV4-dependent calcium influx in endothelial cells. J. Ethnopharmacol. 256:112790. doi: 10.1016/j.jep.2020.112790
Yang, M., Wang, Y., Liang, G., Xu, Z., Chu, C. T., and Wei, H. (2019). Alzheimer’s disease presenilin-1 mutation sensitizes neurons to impaired autophagy flux and propofol neurotoxicity: role of calcium dysregulation. J. Alzheimers Dis. 67, 137–147. doi: 10.3233/JAD-180858
Ye, F., Li, X., Li, F., Li, J., Chang, W., Yuan, J., et al. (2016). Cyclosporin s protects against Lead neurotoxicity through inhibiting mitochondrial permeability transition pore opening in nerve cells. Neurotoxicology 57, 203–213. doi: 10.1016/j.neuro.2016.10.004
Yeh, K. C., Hung, C. F., Lin, Y. F., Chang, C., Pai, M. S., and Wang, S. J. (2020). Neferine, a bisbenzylisoquinoline alkaloid of nelumbo nucifera, inhibits glutamate release in rat cerebrocortical nerve terminals through 5-HT(1A) receptors. Eur. J. Pharmacol. 889:173589. doi: 10.1016/j.ejphar.2020.173589
Yoon, H., Kim, D. S., Lee, G. H., Kim, K. W., Kim, H. R., and Chae, H. J. (2011). Apoptosis induced by manganese on neuronal SK-N-MC cell line: endoplasmic reticulum (ER) stress and mitochondria dysfunction. Environ. Health Toxicol. 26:e2011017. doi: 10.5620/eht.2011.26.e2011017
Yoon, W. S., Yeom, M. Y., Kang, E. S., Chung, Y. A., Chung, D. S., and Jeun, S. S. (2017). Memantine induces NMDAR1-mediated autophagic cell death in malignant glioma cells. J. Korean Neurosurg. Soc. 60, 130–137. doi: 10.3340/jkns.2016.0101.006
Yu, H. M., Wen, J., Wang, R., Shen, W. H., Duan, S., and Yang, H. T. (2008). Critical role of type 2 ryanodine receptor in mediating activity-dependent neurogenesis from embryonic stem cells. Cell Calcium 43, 417–431. doi: 10.1016/j.ceca.2007.07.006
Yu, J., Zhu, C., Yin, J., Yu, D., Wan, F., Tang, X., et al. (2020). Tetrandrine suppresses transient receptor potential cation channel protein 6 overexpression- induced podocyte damage via blockage of RhoA/ROCK1 signaling. Drug. Des. Devel. Ther. 14, 361–370. doi: 10.2147/DDDT.S234262
Yue, C., Soboloff, J., and Gamero, A. M. (2012). Control of type I interferon-induced cell death by Orai1-mediated calcium entry in T cells. J. Biol. Chem. 287, 3207–3216. doi: 10.1074/jbc.M111.269068
Yun, W. J., Zhang, X. Y., Liu, T. T., Liang, J. H., Sun, C. P., Yan, J. K., et al. (2020). The inhibition effect of uncarialin A on voltage-dependent L-type calcium channel subunit alpha-1C: inhibition potential and molecular stimulation. Int. J. Biol. Macromol. 159, 1022–1030. doi: 10.1016/j.ijbiomac.2020.05.100
Yuqi, L., Lei, G., Yang, L., Zongbin, L., Hua, X., Lin, W., et al. (2009). Voltage-dependent anion channel (VDAC) is involved in apoptosis of cell lines carrying the mitochondrial DNA mutation. BMC Med. Genet. 10:114. doi: 10.1186/1471-2350-10-114
Zempel, H., Thies, E., Mandelkow, E., and Mandelkow, E. M. (2010). Abeta oligomers cause localized Ca(2+) elevation, missorting of endogenous tau into dendrites, tau phosphorylation and destruction of microtubules and spines. J. Neurosci. 30, 11938–11950. doi: 10.1523/JNEUROSCI.2357-10.2010
Zhang, E., and Liao, P. (2015). Brain transient receptor potential channels and stroke. J. Neurosci. Res. 93, 1165–1183. doi: 10.1002/jnr.23529
Zhang, X., Yu, L., and Xu, H. (2016). Lysosome calcium in ROS regulation of autophagy. Autophagy 12, 1954–1955. doi: 10.1080/15548627.2016.1212787
Zhao, P., Leonoudakis, D., Abood, M. E., and Beattie, E. C. (2010). Cannabinoid receptor activation reduces TNFalpha-induced surface localization of AMPAR-type glutamate receptors and excitotoxicity. Neuropharmacology 58, 551–558. doi: 10.1016/j.neuropharm.2009.07.035
Zhou, C. (2010). [Inhibition effect of IL-1beta on calcium channels currents in cultured cortical neurons of rat]. Dongwuxue Yanjiu 31, 89–93. doi: 10.3724/sp.j.1141.2010.01089
Zhou, C., Tai, C., Ye, H. H., Ren, X., Chen, J. G., Wang, S. Q., et al. (2006). Interleukin-1beta downregulates the L-type Ca2+ channel activity by depressing the expression of channel protein in cortical neurons. J. Cell Physiol. 206, 799–806. doi: 10.1002/jcp.20518
Zhou, G. H., Zhang, F., Wang, X. N., Kwon, O. J., Kang, D. G., Lee, H. S., et al. (2014). Emodin accentuates atrial natriuretic peptide secretion in cardiac atria. Eur. J. Pharmacol. 735, 44–51. doi: 10.1016/j.ejphar.2014.04.014
Zhu, Y. G., Chen, X. C., Chen, Z. Z., Zeng, Y. Q., Shi, G. B., Su, Y. H., et al. (2004). Curcumin protects mitochondria from oxidative damage and attenuates apoptosis in cortical neurons. Acta Pharmacol. Sin. 25, 1606–1612.
Keywords: calcium ions, transporters, mechanisms, Alzheimer’s disease, review
Citation: Guan P-P, Cao L-L, Yang Y and Wang P (2021) Calcium Ions Aggravate Alzheimer’s Disease Through the Aberrant Activation of Neuronal Networks, Leading to Synaptic and Cognitive Deficits. Front. Mol. Neurosci. 14:757515. doi: 10.3389/fnmol.2021.757515
Received: 12 August 2021; Accepted: 04 November 2021;
Published: 02 December 2021.
Edited by:
Daniela Puzzo, University of Catania, ItalyReviewed by:
Balaji Krishnan, University of Texas Medical Branch at Galveston, United StatesMaud Gratuze, Washington University in St. Louis, United States
Roberto Piacentini, Catholic University of the Sacred Heart, Italy
Copyright © 2021 Guan, Cao, Yang and Wang. This is an open-access article distributed under the terms of the Creative Commons Attribution License (CC BY). The use, distribution or reproduction in other forums is permitted, provided the original author(s) and the copyright owner(s) are credited and that the original publication in this journal is cited, in accordance with accepted academic practice. No use, distribution or reproduction is permitted which does not comply with these terms.
*Correspondence: Pu Wang, wangpu@mail.neu.edu.cn
† These authors have contributed equally to this work