- 1Pediatric Department, “Vittore Buzzi” Children’s Hospital, Milan, Italy
- 2Department of Internal Medicine, University of Pavia, Pavia, Italy
- 3Pediatric unit , Fondazione Istituto di Ricovero e Cura a Carattere (IRCCS) Policlinico S. Matteo and University of Pavia, Pavia, Italy
- 4Pediatric Clinical Research Center “Invernizzi”, Department of Biomedical and Clinical Sciences “L. Sacco”, University of Milan, Milan, Italy
Puberty is a critical phase of life associated with physiological changes related to sexual maturation, and represents a complex process regulated by multiple endocrine and genetic controls. Puberty is driven by hormones, and it can impact the gut microbiome (GM). GM differences between sex emerge at puberty onset, confirming a relationship between microbiota and sex hormones. In this narrative review, we present an overview of precocious pubertal development and the changes in the GM in precocious puberty (PP) in order to consider the role of the sex hormone–gut microbiome axis from the perspective of pediatric endocrinology. Bidirectional interactions between the GM and sex hormones have been proposed in different studies. Although the evidence on the interaction between microbiota and sex hormones remains limited in pediatric patients, the evidence that GM alterations may occur in girls with central precocious puberty (CPP) represents an interesting finding for the prediction and prevention of PP. Deepening the understanding of the connection between the sex hormones and the role of microbiota changes can lead to the implementation of microbiota-targeted therapies in pubertal disorders by offering a pediatric endocrinology perspective.
Introduction
Puberty is a critical phase of life associated with physiological changes related to sexual maturation and represents a complex process regulated by multiple endocrine and genetic controls (1). Gonadal sex hormones are secreted in accordance with the pulsatile secretion of the pituitary gonadotropin follicle-stimulating hormone and luteinizing hormone (FSH and LH), which is activated by the release of the hypothalamic gonadotropin releasing hormone (GnRH) (2). Rising levels of sex steroid hormones trigger changes in physical appearance during pubertal development.
Pubertal development can be at the appropriate time, precocious or delayed. Abnormal pubertal development can cause considerable distress to the patient and could also be a sign of an underlying pathology.
Puberty is driven by hormones, and it can impacts the gut microbiome (GM) (3). Gut microbial communities represent one source of human genetic and metabolic diversity; they influence nutrient acquisition, brain development, immunity, endocrinology and the nervous system (4–6). Few studies have described changes in the gut microbiome as a function of age. It has been observed that the development of the microbiome from infancy to childhood is dependent on multiple factors, and an association between sex hormones and microbiota has been proposed. GM differences between sex emerge at puberty onset, confirming a relationship between microbiota and sex hormones (3, 7). Some theories suggest that the GM regulates the levels of sex hormones via interactions among its metabolites, the immune system, chronic inflammation and some nerve–endocrine axes, such as the gut–brain axis. Additionally, bidirectional interactions between the microbiome and the hormonal system have also been proposed, and the mechanisms of these interactions are being explored; data are limited in pediatrics (8).
In this narrative review, we presented an overview on the precocious pubertal development and the changes in the GM in precocious puberty in order to consider the role of the sex hormone–gut microbiome axis from the perspective of pediatric endocrinology.
Methods
A narrative review was presented (9); a non-systematic summation and analysis of the available literature on the topic of the changes in the GM in precocious puberty and the role of the sex hormone–gut microbiome axis was considered. Authors reviewed the relevant English literature on a specific topic, in the past 15 years, including original papers, metanalysis, clinical trials and reviews. Case reports and case series were excluded. A search in PubMed, Scopus, EMBASE, and Web of Science was adopted. The following search terms (alone and/or in combination) were adopted: precocious puberty, timing of puberty, gut microbiome, sex hormones, sex hormone–gut microbiome axis. The authors revised the abstracts of the available literature (n=83) and reviewed the full texts of potentially relevant articles (n=50) that were analyzed and critically discussed. The reference list of all articles was checked to consider relevant studies. The contributions were independently collected by V.R., G.M., C.R. and C.H., critically analyzed by V.C., S.P. and V.C., S.P., C.B., G.Z discussed the resulting draft before finalizing. The final version was approved by all the coauthors.
Pubertal development
Physiology of the puberty
Puberty is a crucial developmental milestone characterized by the maturation of gametogenesis (precursor cells undergo cell division and differentiation to form mature haploid gametes), the production of gonadal hormones and the development of secondary sexual characteristics and reproductive functions.
Normal puberty results from prolonged, mature activity of the hypothalamic–pituitary–gonadal (HPG) axis (2). The hypothalamus releases the GnRH in a pulsatile way into the pituitary portal venous system, where it stimulates LH and FSH secretion (pulsatile as well). LH primarily stimulates Leydig cells in the testes and theca cells in the ovary to secrete androgens. FSH primarily stimulates the ovarian follicle or seminiferous tubules to form estrogen, inhibin, and eggs or sperm. The interstitial, tubular and follicular compartments act together through paracrine processes to produce estrogen, and regulate sex steroid production and gamete development. Steroid hormones have endocrine negative feedback effects on GnRH and gonadotropin secretion. FSH secretion is suppressed by the negative feedback of inhibin, progesterone, and estradiol. In adult female subjects, critical estradiol concentration stimulates the LH surge that initiates ovulation.
At birth, due to the absence of placental steroids that suppress the HPG axis, there is an activation of such axis, which causes an increased production of steroidal hormones, defining the first step that will be continued in adolescence. This transient activation starts approximately one week after birth, and it stops after few months (approximately 6 months of age) (10–12).
The HPG axis is not completely dormant throughout childhood, especially in females, who show moderately higher FSH concentrations than males. Sometimes, it is also possible to see ovarian follicles using ultrasounds. During adolescence, the HPG axis undergoes complete reactivation.
The most important GnRH-inhibitory systems are gabaergic (neurons that produce gamma-aminobutyric acid) and opioidergic; Kisspeptin, neurokinin B and dynorphin A, three neuropeptides present in the arcuate nucleus (ARC), are considered to be fundamental generators that influence GnRH release, as they contribute significantly to the physiology of puberty in boys and girls (2).
The role of leptin in the physiology of puberty is well known. Leptin is a cytokine produced mainly by adipocytes, which acts as an anorectic factor, playing an essential role in controlling body weight, food intake, and energy balance by inhibiting the hypothalamic neuropeptide Y (NPY), thus suppressing appetite (13, 14). Normal body weight and composition must be attained during childhood to avoid pubertal dysfunction (1). In addition to the leptin-NPY interaction, some studies showed that leptin acts on puberty and reproductive function by directly interacting with the KiSS-1gene. GnRH neurons lack leptin receptors, but KiSS-1 neurons express them. Leptin directly stimulates kisspeptin release and mediates the pulsatile release of GnRH (15).
Physical changes occurring in puberty in male and female adolescents and the assessment of secondary sexual characteristics (breast buds in girls, testicular volume in boys, pubic hair in both), were classified according to the Marshall and Tanner classification
Precocious puberty
Puberty is a complex process with wide physiological variation. Mechanisms regulating the onset of puberty involve genetic, nutritional and environmental interactions (1).
Abnormal fetal nutrition along with the endocrine system could lead to developmental alterations that permanently affect structure, physiology and metabolism. Interactions between hormones and nutrition during crucial periods of growth are essential concerning metabolic adaptation response control and pubertal development expectation (16).
An increasing amount of evidence suggests that the prenatal and early postnatal periods represent an important period for the programming of puberty onset (17). Various studies have shown that prenatal exposure to unfavorable environmental factors, such as factors responsible for children born SGA (small for gestational age) and/or IUGR (intrauterine growth restriction), have an effect on puberty timing (18, 19). A child born SGA may undergo several puberty alterations, such as precocious puberty (20–22).
Precocious puberty (PP) is defined by the early appearance of secondary sexual characteristics before the age of 8 years in female adolescents and 9 years in males (23). According to the underlying physiopathological process, pathological PP is classified as follows:
• Central precocious puberty (CPP) or gonadotropin-dependent PP (or true precocious puberty) caused by an early maturation of the HPG axis due to congenital or acquired central nervous system (CNS) lesions or monogenic defects, or it can be idiopathic (1);
• Peripheral precocious puberty (PPP) or gonadotropin-independent PP (or pseudoprecocious puberty), due to an excessive secretion of gonadal sex hormones or adrenal hormones from a genetic or tumoral etiology, germ cell tumors secreting hCG (human chorionic gonadotropin—exclusively in boys), or an exogenous source (1).
It has been evaluated that CPP affects 1 in 5000-10000 children, and is 10 times more common in females than in males (24). In addition, most of the cases of CPP in females seem to be idiopathic (25), whereas a higher prevalence of CPP in males seems to be commonly caused by pathological brain lesions (26). In particular, hypothalamic hamartoma is the most common brain lesion causing CPP.
There are some reports of familial forms, but the genetic basis is not completely understood. Some studies have shown associations with mutations (loss or gain of function) of KISS1 and makorin ring finger (MRF3) genes and their receptors. Mutations in these genes result in CPP; on the contrary, in familial CPP, MKRN3 defects were found in approximately 30% of families in subjects with apparently sporadic CPP, and MKRN3 defects were noted in approximately 8% of cases (27).
Patients with PP show accelerated sexual and physical growth concurrently with a growth spurt. If untreated, the accelerated epiphysial growth could lead to a short stature in adulthood due to premature epiphysial closure.
An accurate personal and familiar history, a complete physical examination, hormonal, and radiological exams is crucial in the PP diagnosis (23, 24, 28, 29).
The clinical examination should be focused on the auxological data, the assessment of pubertal signs according to the Marshall and Tanner classification (12, 30), the growth pattern during the last 6–12 months, the rate of progression of pubertal signs and additional signs of puberty (acne, oily skin, erections, nocturnal emissions in boys and vaginal discharge and menstrual bleedings in girls).
The baseline LH level is a promising biomarker to diagnose CPP (31); a basal morning LH value of more than 0.2 mUI/ml is usually considered indicative of puberty (28, 31–37). In addiction, an LH to FSH ratio higher than 0.6 has been associated with CPP (31, 34). Moreover, the GnRH stimulation test remains the gold standard to identify CPP, and the cutoff peak LH level of >5 IU/L is widely used to diagnose CPP (come sopra).
Other hormonal evaluations should include thyroid tests, testosterone, estradiol, 17-hydroxyprogesterone (17-OHP), carcinoembryonic antigen (CEA), Cancer antigen 125 (CA125), alpha-fetoprotein and beta-hCG depending on the patient’s history (38).
To define the biological age of the child, a bone age X-ray of the nondominant (left) hand and wrist is taken. An advanced bone age of more than 2.5 standard deviations (SD) or more than 2 years is more likely associated with pathological PP (28, 35).
In girls, pelvic ultrasound is a useful tool to assess the premature pubertal development of ovaries and exclude the presence of ovarian cysts or tumors (39, 40).
Brain MRI is suggested in patients with a CPP diagnosis to rule out CNS lesions (28), that should be performed routinely in young boys (< 6 years) (28).
The most important goals of the PP treatment are to preserve the adult height and to reduce the associated psychosocial stress.
GnRH agonists, with 1-month or 3-month depot formulations, are the standard of care in CPP. GnRH agonist therapy is widely considered safe. The most common adverse reactions include local skin effects and postmenopausal symptoms (35). The periodic verification of pubertal progression, growth velocity, and skeletal maturation is required.
The treatment of PPP varies according to the pathogenesis, and the primary aim of treatment is to eliminate the endogenous or exogenous sources of sex steroids (41, 42). Surgery is indicated in adrenal and gonadal tumors.
Gut–sex hormones axis
The human intestinal tract is colonized by a large number of microorganisms (circa 1013-1014), known together as “microbiota”. The gut microbiome is the human body’s major ecosystem, so much so that according to some authors, it represents a “separate organ” (43). The human colon can be populated by more than 1000 different bacterial species and, each host can boast at least 160, which, however, vary in type and quantity depending on the person’s own health (44–46). This element underscores the interdependence that exists between host and GM, which affects multiple aspects of host health, particularly endocrine, gastroenterological (digestive function and intestinal permeability), and immune (resistance to foreign pathogens and stimulation of immunity) (47). Microbiota interact with a variety of metabolic and endocrine pathways of the host through genetic expression of more than 100 times the human genome. GM’s variety, composition and impact on health depend on a great number of variables, both internal, such as age, genetic factors, gender, and endocrine and immune systems, as well as external factors, such as diet, environment, drugs, and pathogens. All together they influence the delicate balance of the intestinal microecological system. In addition, research has shown that an imbalance in the GM can lead to a range of related diseases, especially those of autoimmune origin (5, 47).
In healthy subjects, more than 90% of bacteria are part of Firmicutes or Bacteroides’ phyla, and alterations in microbiota diversity are related to adverse outcomes in the host’s health. A decreased Firmicutes/Bacteroides ratio correlates with health issues such as obesity and immunological diseases (4, 48). Some authors have reported an association between an incremented Firmicutes/Bacteroides ratio and type 1 diabetes mediated by cell junction disruption with incremental gut permeability, bacterial translocation and the subsequent expression of pro-inflammatory cytokines (49).
The relationship between sex hormones and GM has been widely explored in recent studies and is an expanding research field that may lead to new therapeutic options for a great variety of sex-related diseases; the cluster of the gut microbiome’s genes capable of influencing sex hormone levels has been named the “microgenderome”.
One of the earliest studies was performed at the cellular level in the 1980s, in which it was found that progesterone promoted the growth of Bacteroides species and Prevotella intermedia (50, 51). Recently, Yurkovetskiy et al. (52) sequenced bacterial DNA extracted from the cecal contents of prepubertal (4 weeks old) and postpubertal (10-13 weeks old) mice and found that α-diversity was not significantly different between the sexes in prepubertal mice, which was evident among postpubertal mice. Furthermore, by sequencing the 16S rRNA genes of the microbiota of males, females, and castrated males, they observed that the microbiome of females was closer to that of castrated males than that of uncastrated males (50, 52). Among the most recent studies evaluating the relationship between microbiota and sex hormones, Elin Org et al. (53) further demonstrated the effect of androgens on microbiota composition, particularly by assessing the effects arising from gonadectomy and hormone supplementation (53). In contrast, there are still few studies conducted in humans evaluating the relationship between estrogen fluctuation and gut microbiome composition (4, 48). Moreover, these studies have an important limitation dictated by interfering factors, such as genetics and the environment, so most results can only support the existence of a correlation between sex hormones and the microbiome, rather than a causal relationship (50). Koren et al. (54), when sequencing stool samples from 91 women, observed that the gut microbiome was markedly altered during pregnancy, especially during the third trimester, when estrogen peaks, regardless of health status (50, 54). A European study by Mueller et al. (55) showed that healthy males had a higher abundance of Bacteroides-Prevotella than fertile females, while the microbiota of postmenopausal women did not differ from that of males (50, 56). Both studies demonstrate how estrogen and related female hormones are crucial in regulating the composition of the gut microbiome.
Therefore, it is well known that microbiota can affect estrogen levels and that, in turn, estrogen levels may be influenced by microbiota composition and diversity. Microbiome is capable of metabolizing estrogens via the expression of B-glucuronidase, an enzyme that mediates the deconjugation of dietary and non-dietary estrogen. Unconjugated estrogen can enter the systemic flow and become metabolically active by acting on alpha and beta estrogen receptors, which are expressed in a variety of organs and tissues; estrogen activity has an impact not only on reproductive health but also on cardiovascular risk, metabolic and bone homeostasis and the central nervous system (49, 50). Microbiota diversity is associated with higher urinary estrogen levels in postmenopausal women and in men, whereas in premenopausal women, estrogen levels do not seem to be influenced by microbiota composition, suggesting that microbiota mostly influences the levels of non-ovarian estrogens. The supplementation of phytoestrogen is capable of promoting gut colonization from specific bacterial species, and a phytoestrogen-rich diet may be associated with a lower risk of metabolic syndrome in Asian postmenopausal women (57, 58).
As already stated, the microbiome also influences the level of androgens in the host’s organism, and this might occur through a similar mechanism to that observed in women; a recent study found the levels of non-glucuronidated dihydrotestosterone to be lower in the distal intestine of germ-free mice compared to mice with normal microbiota, thus suggesting that intestinal bacteria express genes capable of metabolizing human sex hormones (59).
In turn, the sex hormone level may also affect microbiome composition: androgen excess, as in PCOS patients, may also lead to dysbiosis and lower bacterial diversity. PCOS is an endocrine disease characterized by higher androgen and lower estrogen levels, and several studies associated intestinal dysbiosis in PCOS patients with lower bacterial diversity, resulting in reduced butyrate production, higher BMIs and higher testosterone serum concentrations. Additionally, the gut microbiome plays an important role in determining insulin secretion by producing SCFAs, which help to reduce the inflammatory response; dysbiosis may lead to insulin resistance and alterations in glucose metabolism, as in polycystic ovary syndrome; higher insulin levels stimulate the ovary in producing androgens, thus perpetuating the pathogenetic mechanism of PCOS (48).
Microbiota and their metabolites may also affect every stage of female fertility, pregnancy, embryo development and timing of delivery, by colonizing the vaginal tract and, according to some authors, the endometrium and placenta. Microbiota’s alterations have been associated with the secretion of proinflammatory cytokines and preterm delivery (60). Neonates born from cesarean section delivery show lower diversity in intestinal bacteria, probably because they have not been colonized by maternal intestinal flora by passage through the vaginal tract (48).
Another possible mechanism for explaining microbiota’s influence on sex hormone levels involves the “gut-brain axis”, the two-way communication pathway between gut and CNS, according to which gut bacteria are an important mediator between the brain and the endocrine system (4). GM is central in modulating the brain–gut axis, and the gut barrier SCFAs, such as acetate, propionate, and butyrate, besides being modulators of inflammation capable of regulating gut motility and wound healing, represent a link between the microbiome and the gut–brain axis (4, 5, 61–63). In addition, the incidence of functional gastrointestinal disorders, such as functional dyspepsia and irritable bowel syndrome, resulting in impaired motility and/or altered sensitivity, are significantly higher in females, presumably due to a complex interaction between sex hormone signaling and stress reactivity in brain–gut axis function (6, 64). Specifically, estrogens have been observed to interfere with gastrointestinal motility and sensitivity through the direct activation of their receptors, located throughout the brain–gut axis, and indirectly through the modulation of other receptor systems (6). Gastrointestinal motility is reduced in women during the follicular phase of the ovarian cycle, when estrogen levels are high (6, 65). Furthermore, supporting the hypothesis that circulating female hormones play an important role in delayed gastric emptying, hormone replacement therapy administered to pre- and postmenopausal women correlates with a slower rate of gastric emptying than that of postmenopausal women not receiving hormone therapy, which in turn is similar to that of men of the same age (66). In contrast, testosterone, or androgens in general, appear to have no effect on gastric motility or gastric hypersensitivity (67, 68).
Estrogens implement their long-term mechanism of action through actions on nuclear receptors and rapid, nongenomic action through the activation of estrogen receptor 1 (ER 1) receptors coupled to membrane G proteins (6, 6). ERs are ubiquitously expressed in the CNS and in pathways involved in visceral pain perception, including the hypothalamus, amygdala, and midbrain, all of which have been shown to send extensive projections to vagal neurons involved in the modulation of gastrointestinal function (6, 69–71). Specifically, in peripheral visceral afferents, estrogen appears to modulate nociception by altering the opening of ion channels and the regulation of receptor expression, as well as activating the cholic tachykinin neurokinin 1 receptor and inducing the release of substance P (6, 72, 73). In addition to interfering with pain modulation, finally, estrogen is involved in visceral information processing in the CNS. Cerebral imaging studies have found that, in comparison with males with IBS, females with IBS display a greater activation of emotional circuits, including the amygdala and locus coeruleus, in response to adverse visceral stimuli (74).
Furthermore, several studies have demonstrated better cognitive functions and reductions in psychiatric symptoms in selected patients treated with fecal transplant. More studies on humans are needed to better understand the underlying mechanisms of this axis (4, 75).
In Figure 1, the sex hormone–gut microbiome axis is shown.
Microbiome in physiological pubertal development
It is well known how a gradual change in microbiota composition occurs with age with a general reduction in the number of aerobes and facultative anaerobes and an increase in the populations of obligate anaerobic species. Traditionally, the common idea is that between one and two years of age, the human GM starts to resemble that of an adult, which is dominated by species from phyla Firmicutes, Bacteroidetes, Proteobacteria, and Actinobacteria. However, many differences were observed at the genus level between adolescent and adult fecal microbiota (3, 7).
Agans et al. (7) conducted a study to assess the distal GM of adolescent children, which showed that abundance of members of Bifidobacterium and Clostridium genera, species known to colonize the newborn gut and to decrease gradually between 2 and 18 years of life, was statistically significantly higher in adolescent children than adults. The prevalence of these genera had not been recognized previously among preadolescent and adolescent age groups (7).
In 2020, Yuan et al. (76)conducted a cross-sectional study analyzing the biodiversity of the GM at different puberty stages (5-15 years) through 16S rRNA sequencing. No difference in alpha or beta diversity between non-pubertal and pubertal subjects was found, but the study evidenced differential bacterial taxa between the two groups. In particular, non-pubertal subjects were characterized by mainly microorganisms belonging to the order Clostridiales, family Costridiaceae, and genus Coprobacillus. On the other hand, the puberal group showed a higher prevalence of class Betaproteobacteria, order Burkhollderiales (76). Further analyses of the association between serum sex hormones and bacterial abundance were conducted. The results highlight that the level of testosterone was associated with the abundance of Adlercreutzia, Dorea, Clostridium and Parabacteroides genera. Authors hypothesized that these bacteria might be affected by sex hormones (76). Several studies have investigated the connection between gut microbes and sex steroid hormones. Shin et al. (77) demonstrated a relationship between intestinal bacterial community profiles and testosterone/estrogen status in humans: Acinetobacter, Dorea, Ruminococcus and Megamonas were significantly correlated with testosterone levels, while Slackia and Butyricimonas correlated significantly with estradiol levels (77).
It has been reported that GM does not seem to be affected by gender in children, but differences emerge at puberty onset (59). Microbiota-related diseases also show a gender bias, supporting that the relationship between intestinal bacteria and gender may be biunivocal (4). Although data on adolescents’ GM are still limited, a recent cross-sectional survey found that the distinction of the GM between the two sexes becomes more marked at puberty (78, 79). Comparing teens’ and adults’ microbiota, it was found that the amounts of bifidobacteria, in particular, decreased with age in several studies (7, 80–82), and age-related associations with Bacteroidetes and Firmicutes (80–82)were also reported (79). Hollister et al. (81) compared puberal and adult GM composition, pointing out that, during pubertal development, GM in girls changes progressively, increasingly resembling that of adult women, directly proportional to their pubertal developmental degree. In both males and females, dominant taxa are Clostridia and Bacteroidia, and the major element suggesting maturation of microbiota is represented by a change in the dominance of the Clostridiales and Bacteroidales classes of bacteria. Indeed, during puberty, the relative abundance of Clostridiales gradually increases and that of Bacteroidales decreases, gradually resembling the composition of the adult microbiota. In terms of phylum, the abundance of Firmicutes increased as puberty progressed, while the abundance of Bacteroidetes decreased. However, although Hollister et al. (81) observed GM changes in both boys and girls, only in female adolescents statistically significant data were obtained, reasonably due to later males’ pubertal development (81).
These data were confirmed by Yuan et al. (78) who determined the characteristics of the GM of both genders at different pubertal status. The GM was analyzed in 89 Chinese participants aged 5–15 years. Participants were divided into prepuberty and puberty groups for both males (n=49) and females (n=40). This cross-sectional study revealed that sex differences in the GM composition and predicted metabolic profiles existed before puberty and it became more significant in puberty. Specifically, results indicate that Dorea, Megamonas, Bilophila, Parabacteroides and Phascolarctobacterium genera represent microbial markers for pubertal subjects (78). They suggested that sex-dependent GM diversity is, in part, due to sex hormones, and, in part, to other non-hormonal influencing factors (78).
Gut microbioma and precocious puberty
Considering evidences on the role of the GM during physiological pubertal development, their role in pathological puberty is becoming of increasing interest. As reported (83), timing of puberty can be influenced by GM, particularly by certain Clostridia species, including species of the genera Ruminococcaceae Faecalibacterium and Ruminococcus, which regulate host sex hormone levels. Specifically, these species affect estrogen metabolism through their beta-glucuronidase activity (81). The beta-glucuronidase enzymes of Ruminococcus and Faecalibacterium spp. are able to cleave both estrone and estradiol, whereas Bacteroides species are only capable of metabolizing estrone. Therefore, estrone-estrogen metabolite ratio in urine correlates positively with the relative abundance of Ruminococcus and negatively with that of Bacteroides spp (81, 84). According to these data, it is conceivable that the GM may partly regulate the onset of puberty through its estrogen metabolism. However, as much as the GM, through specific gut microbes capable of metabolizing estrogen, seems capable of regulating puberty, the reverse may also be possible. In fact, sex hormones could directly affect the growth of specific taxa by directing the maturation of the gut microbiota (81).
Furthermore, recent studies have observed that metabolites produced by the GM could influence the human endocrine system, activating the enteric nervous system. Some of the best studies on microbiota functions highlight how gut microorganisms provide energy to the host through the production of short-chain fatty acids (SCFAs), including butyrate (the most abundant SCFA) and propionate, both of which participate in bile salts metabolism and play an important role in brain-gut axis (4, 48). It has been demonstrated that Free Fatty Acid Receptor (FFAR) 2 and FFAR3, endogenous receptors, interact with the SCFAs and have been shown to be expressed in enteroendocrine cells that produce peptide YY, an anorectic hormone, with consequent involvement in the regulation of the host energy, appetite, adipose tissue stores and hormonal balance, influencing puberty timing (85, 86)
Indeed a close association between obesity and puberty has been found; in particular, PP has been positively related with body mass index (BMI). On the basis of this information and given that children affected by PP tend to be obese, it has been hypothesized that GM could be involved in the pathogenesis of PP. The study conducted by Dong et al. (87) elucidated differences in the GM between patients with idiopathic central precocious puberty (ICPP) (n=25) and healthy girls (n=23). Authors applied 16S rDNA sequencing to compare the GM between two groups. They observed that the gut genera identified in ICPP are similar to those that are associated with obesity, in particular, Rumicoccus Gemmiger, Oscillibacter and Clostridium XIVb. Considering microbial species levels, girls with ICPP were enriched in Rumicoccus bromii, Ruminococcus gnavus, and Ruminococcus leptum. The first two were found in obese populations; they could promote the energy absorption and hyperplasia of adipose tissue, while Ruminococcus leptum was reported to influence human weight changes (87–89). These results highlight the association among obesity, ICPP and GM dysbiosis. The authors hypothesized that GM dysbiosis leads preadolescent girls to a process similar to that in obese patients and that the proliferation and deposition of adipocytes trigger precocious puberty. However, intestinal dysbiosis could also induce the earlier activation of the hypothalamic–pituitary–gonadal axis (HPGA) (87).
The involvement of the GM in the mechanism of secretion of estrogen, FSH and LH has been investigated in different studies, but it is still unclear. A previous study indicated a relationship between estrogen and bacteria, such as Clostridia and Ruminococcaceae, and a significant microbial differences among the control group and ICPP (90). Dong et al. (87) explored the relationship between three clinical biomarkers (FSH, LH and insulin resistance) and the GM. Considering ICPP girls, authors demonstrated a positive correlation between FSH and Fusobacterium, and LH and Gemmiger, and a negative correlation between LH and Romboutsia (87). In addition, insulin resistance has been positively correlated with Gemmiger, Ruminococcus, Megamonas and Bifidobacterium (87).
In female puberty onset, the important role of leptin is well known. Leptin is an adipocyte metabolic peptide, and the gene involved in its expression is correlated with SCFAs (83, 85, 88, 91, 92).
The close association among the GM, hormone secretion and obesity inspired the study of the mechanism of the GM in triggering CPP. ICPP girls investigated in the work by Dong et al. were characterized by microbes associated with SCFA production: Ruminococcus bromii, Ruminococcus callidus, Roseburia inulinivorans, Coprococcus eutactus, Clostridium sporosphaeroides and Clostridium lactatifermentans. The relationship between SCFA production and ICPP is explained through the mechanism induced by a high concentration of SCFAs to the expression of the leptin gene, which activates the HPGA, which, consequently, leads to the onset of puberty (87).
Li et al. (93) enrolled 27 CPP girls, 24 overweight girls and 22 healthy controls to explore the connection between obesity and CPP. This study showed that CPP patients exhibited overrepresented Alistipes, Klebsiella and Sutterella, which are normally present in patients with neurological diseases. These microorganisms produce metabolites with neurotransmission activity (serotonin and dopamine), which trigger the earlier onset of puberty activating HPGA. The authors identified Prevotella both in CPP and in the overweight group; branched-chain amino acid production could promote insulin resistance. This mechanism could explain the high occurrence of obesity in CPP patients (93). In addition, in both groups, elevated nitric oxide synthesis was observed, which is an important gas neurotransmitter that stimulates the secretion of gonadotropin-releasing hormone and promote insulin resistance (93). These conditions, the altered expression of the GM, could explain the link between CPP and obesity (93), as shown in Figure 2.
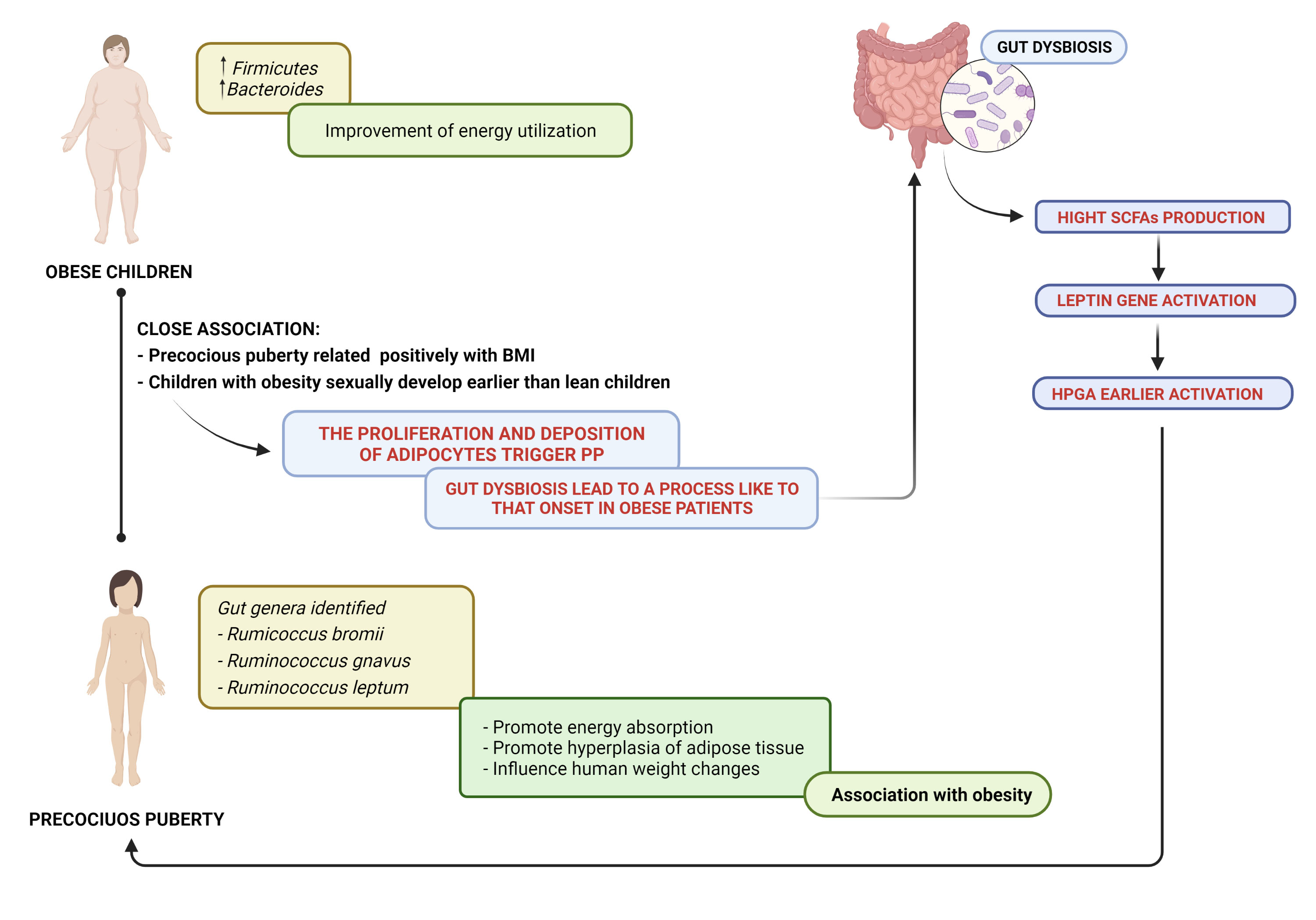
Figure 2 Precocious puberty–gut microbiome and obesity: a close association. SCFAs, short-chain fatty acids; HPGA, hypothalamic–pituitary–gonadal axis.
As reported (1), the macronutrient food content, such as high fat intake, may modulate the premature activation of the HPG axis, inducing precocious activation of puberty. Recently, in experimental model Bo et al. (94) showed that the effect of high-fat diet (HFD) on precocious puberty is regulated by the interaction of gut microbiota and hormones. HFD after weaning caused PP, increased serum estradiol, leptin, deoxycholic acid and GnRH in the hypothalamus (94). In particular, GnRH was positively correlated with Desulfovibrio, Lachnoclostridium, GCA-900066575, Streptococcus, Anaerotruncus, and Bifidobacterium, suggesting that these bacteria may have a role in promoting sexual development (94). Additionally, the authors (94) reported that “HFD-microbiota” transplantation promoted the PP of mice, supporting that GM modulates local and systemic levels of sex steroids promoting precocious puberty.
Conclusions
Bidirectional interactions between the GM and the sex hormones have been proposed in different studies. During puberty, the somatic developmental changes are predominantly driven by hormones; therefore, this dynamic and transitional period represents an opportunity to assess the impact of potential hormonal effects on the GM. Although the evidence of the interaction between microbiota and sex hormones remains limited in pediatric patients, the evidence that diversity of the GM at different puberty stages exists and that GM alterations may occur in girls with CPP represents an interesting finding for the prediction and prevention of precocious pubertal development. Deepening the understanding of the connection between the sex hormones and the role of microbiota changes can lead to the implementation of microbiota-targeted therapies in pubertal disorders by offering a pediatric endocrinology perspective.
Author contributions
VC, VR, GM, CR, CH, SP, CB, GZ participated in the study design, project management, and supervision. VC, VR, GM, CR, CH were responsible for the conceptualization and design of forms, data management, writing, and editing. VC, SP, CB, GZ supervised the manuscript. All authors were contributed to this article and approved the submitted version.
Conflict of interest
The authors declare that the research was conducted in the absence of any commercial or financial relationships that could be construed as a potential conflict of interest.
Publisher’s note
All claims expressed in this article are solely those of the authors and do not necessarily represent those of their affiliated organizations, or those of the publisher, the editors and the reviewers. Any product that may be evaluated in this article, or claim that may be made by its manufacturer, is not guaranteed or endorsed by the publisher.
References
1. Calcaterra V, Verduci E, Magenes VC, Pascuzzi MC, Rossi V, Sangiorgio A, et al. The role of pediatric nutrition as a modifiable risk factor for precocious puberty. Life (Basel) (2021) 11:1353. doi: 10.3390/life11121353
2. Rosenfield RL, Cooke DW, Radovick S. Puberty and its disorders in the female. In: Pediatric endocrinology. (Amsterdam:Elsevier) (2014) p. 569–663.e1.
3. Markle JGM, Frank DN, Mortin-Toth S, Robertson CE, Feazel LM, Rolle-Kampczyk U, et al. Sex differences in the gut microbiome drive hormone-dependent regulation of autoimmunity. Science (2013) 339:1084–8. doi: 10.1126/science.1233521
4. Yoon K, Kim N. Roles of sex hormones and gender in the gut microbiota. J Neurogastroenterol Motil (2021) 27:314–25. doi: 10.5056/jnm20208
5. Tremaroli V, Bäckhed F. Functional interactions between the gut microbiota and host metabolism. Nature (2012) 489:242–9. doi: 10.1038/nature11552
6. Jiang Y, Greenwood-Van Meerveld B, Johnson AC, Travagli RA. Role of estrogen and stress on the brain-gut axis. Am J Physiol Gastrointest Liver Physiol (2019) 317:G203–9. doi: 10.1152/ajpgi.00144.2019
7. Agans R, Rigsbee L, Kenche H, Michail S, Khamis HJ, Paliy O. Distal gut microbiota of adolescent children is different from that of adults. FEMS Microbiol Ecol (2011) 77:404–12. doi: 10.1111/j.1574-6941.2011.01120.x
8. Carabotti M, Scirocco A, Maselli MA, Severi C. The gut-brain axis: Interactions between enteric microbiota, central and enteric nervous systems. Ann Gastroenterol (2015) 28:203–9.
9. Gregory AT, Denniss AR. An introduction to writing narrative and systematic reviews — tasks, tips and traps for aspiring authors. Heart Lung Circ (2018) 27:893–8. doi: 10.1016/j.hlc.2018.03.027
10. Waterland RA, Jirtle RL. Early nutrition, epigenetic changes at transposons and imprinted genes, and enhanced susceptibility to adult chronic diseases. Nutrition (2004) 20:63–8. doi: 10.1016/j.nut.2003.09.011
11. Farello G, Altieri C, Cutini M, Pozzobon G, Verrotti A. Review of the literature on current changes in the timing of pubertal development and the incomplete forms of early puberty. Front Pediatr (2019) 7:147. doi: 10.3389/fped.2019.00147
12. Marshall WA, Tanner JM. Variations in pattern of pubertal changes in girls. Arch Dis Child (1969) 44:291–303. doi: 10.1136/adc.44.235.291
13. Topaloglu AK, Reimann F, Guclu M, Yalin AS, Kotan LD, Porter KM, et al. TAC3 and TACR3 mutations in familial hypogonadotropic hypogonadism reveal a key role for neurokinin b in the central control of reproduction. Nat Genet (2009) 41:354–8. doi: 10.1038/ng.306
14. Iwasa T, Matsuzaki T, Kinouchi R, Gereltsetseg G, Murakami M, Nakazawa H, et al. Changes in the responsiveness of serum leptin and hypothalamic neuropeptide y MRNA levels to food deprivation in developing rats. Int J Dev Neurosci (2011) 29:377–80. doi: 10.1016/j.ijdevneu.2011.03.006
15. Smith JT, Acohido BV, Clifton DK, Steiner RA. KiSS-1 neurones are direct targets for leptin in the Ob/Ob mouse. J Neuroendocrinol (2006) 18:298–303. doi: 10.1111/j.1365-2826.2006.01417.x
16. Schlaudecker EP, Munoz FM, Bardají A, Boghossian NS, Khalil A, Mousa H, et al. Small for gestational age: Case definition & guidelines for data collection, analysis, and presentation of maternal immunisation safety data. Vaccine (2017) 35:6518–28. doi: 10.1016/j.vaccine.2017.01.040
17. Wu Y, Sánchez BN, Goodrich JM, Dolinoy DC, Cantoral A, Mercado-Garcia A, et al. Dietary exposures, epigenetics and pubertal tempo. Environ Epigenet (2019) 5:dvz002. doi: 10.1093/eep/dvz002
18. Clayton PE, Cianfarani S, Czernichow P, Johannsson G, Rapaport R, Rogol A. Management of the child born small for gestational age through to adulthood: A consensus statement of the international societies of pediatric endocrinology and the growth hormone research society. J Clin Endocrinol Metab (2007) 92:804–10. doi: 10.1210/jc.2006-2017
19. Abreu AP, Kaiser UB. Pubertal development and regulation. Lancet Diabetes Endocrinol (2016) 4:254–64. doi: 10.1016/S2213-8587(15)00418-0
20. Koziel S, Jankowska EA. Effect of low versus normal birthweight on menarche in 14-Year-Old polish girls. J Paediatr Child Health (2002) 38:268–71. doi: 10.1046/j.1440-1754.2002.00793.x
21. Adair LS. Size at birth predicts age at menarche. Pediatrics (2001) 107:e59. doi: 10.1542/peds.107.4.e59
22. Sloboda DM, Hart R, Doherty DA, Pennell CE, Hickey M. Age at menarche: Influences of prenatal and postnatal growth. J Clin Endocrinol Metab (2007) 92:46–50. doi: 10.1210/jc.2006-1378
23. Lebrethon MC, Bourguignon JP. Management of central isosexual precocity: Diagnosis, treatment, outcome. Curr Opin Pediatr (2000) 12:394–9. doi: 10.1097/00008480-200008000-00020
24. Cesario SK, Hughes LA. Precocious puberty: A comprehensive review of literature. J Obstet Gynecol Neonatal Nurs (2007) 36:263–74. doi: 10.1111/j.1552-6909.2007.00145.x
25. Prété G, Couto-Silva A-C, Trivin C, Brauner R. Idiopathic central precocious puberty in girls: Presentation factors. BMC Pediatr (2008) 8:27. doi: 10.1186/1471-2431-8-27
26. Choi KH, Chung SJ, Kang MJ, Yoon JY, Lee JE, Lee YA, et al. Boys with precocious or early puberty: Incidence of pathological brain magnetic resonance imaging findings and factors related to newly developed brain lesions. Ann Pediatr Endocrinol Metab (2013) 18:183–90. doi: 10.6065/apem.2013.18.4.183
27. Silveira LG, Noel SD, Silveira-Neto AP, Abreu AP, Brito VN, Santos MG, et al. Mutations of the KISS1 gene in disorders of puberty. J Clin Endocrinol Metab (2010) 95:2276–80. doi: 10.1210/jc.2009-2421
28. Latronico AC, Brito VN, Carel J-C. Causes, diagnosis, and treatment of central precocious puberty. Lancet Diabetes Endocrinol (2016) 4:265–74. doi: 10.1016/S2213-8587(15)00380-0
29. Carel J-C, Léger J. Clinical practice. precocious puberty. N Engl J Med (2008) 358:2366–77. doi: 10.1056/NEJMcp0800459
30. Marshall WA, Tanner JM. Variations in the pattern of pubertal changes in boys. Arch Dis Child (1970) 45:13–23. doi: 10.1136/adc.45.239.13
31. Brito VN, Spinola-Castro AM, Kochi C, Kopacek C, Silva da PCA, Guerra-Júnior G. Central precocious puberty: Revisiting the diagnosis and therapeutic management. Arch Endocrinol Metab (2016) 60:163–72. doi: 10.1590/2359-3997000000144
33. Calcaterra V, Klersy C, Vinci F, Regalbuto C, Dobbiani G, Montalbano C, et al. Rapid progressive central precocious puberty: Diagnostic and predictive value of basal sex hormone levels and pelvic ultrasound. J Pediatr Endocrinol Metab (2020) 33:785–91. doi: 10.1515/jpem-2019-0577
34. Cantas-Orsdemir S, Eugster EA. Update on central precocious puberty: From etiologies to outcomes. Expert Rev Endocrinol Metab (2019) 14:123–30. doi: 10.1080/17446651.2019.1575726
35. Aguirre RS, Eugster EA. Central precocious puberty: From genetics to treatment. Best Pract Res Clin Endocrinol Metab (2018) 32:343–54. doi: 10.1016/j.beem.2018.05.008
36. Yeh S-N, Ting W-H, Huang C-Y, Huang S-K, Lee Y-C, Chua W-K, et al. Diagnostic evaluation of central precocious puberty in girls. Pediatr Neonatol (2021) 62:187–94. doi: 10.1016/j.pedneo.2020.12.001
37. Bangalore Krishna K, Fuqua JS, Rogol AD, Klein KO, Popovic J, Houk CP, et al. Use of gonadotropin-releasing hormone analogs in children: Update by an international consortium. Horm Res Paediatr (2019) 91:357–72. doi: 10.1159/000501336
38. Berberoğlu M. Precocious puberty and normal variant puberty: Definition, etiology, diagnosis and current management - review. Jcrpe (2009) 1:164–74. doi: 10.4274/jcrpe.v1i4.3
39. Mazgaj M. Sonography of abdominal organs in precocious puberty in girls. J Ultrason (2013) 13:418–24. doi: 10.15557/JoU.2013.0044
40. Stranzinger E, Strouse PJ. Ultrasound of the pediatric female pelvis. Semin Ultrasound CT MRI (2008) 29:98–113. doi: 10.1053/j.sult.2007.12.002
41. Schoelwer M, Eugster EA. Treatment of peripheral precocious puberty. Endocrine Dev (2016) 29:230–9. doi: 10.1159/000438895
42. Qudsiya Z, Gupta V. Precocious pseudopuberty. In: StatPearls. Treasure Island (FL: StatPearls Publishing (2022).
43. Possemiers S, Bolca S, Verstraete W, Heyerick A. The intestinal microbiome: A separate organ inside the body with the metabolic potential to influence the bioactivity of botanicals. Fitoterapia (2011) 82:53–66. doi: 10.1016/j.fitote.2010.07.012
44. MetaHIT Consortium, Qin J, Li R, Raes J, Arumugam M, Burgdorf KS, et al. A human gut microbial gene catalogue established by metagenomic sequencing. Nature (2010) 464:59–65. doi: 10.1038/nature08821
45. Sommer F, Bäckhed F. The gut microbiota — masters of host development and physiology. Nat Rev Microbiol (2013) 11:227–38. doi: 10.1038/nrmicro2974
46. Fukuda S, Ohno H. Gut microbiome and metabolic diseases. Semin Immunopathol (2014) 36:103–14. doi: 10.1007/s00281-013-0399-z
47. Blumberg R, Powrie F. Microbiota, disease, and back to health: A metastable journey. Sci Transl Med (2012) 4(137):137rv7. doi: 10.1126/scitranslmed.3004184
48. Qi X, Yun C, Pang Y, Qiao J. The impact of the gut microbiota on the reproductive and metabolic endocrine system. Gut Microbes (2021) 13:1–21. doi: 10.1080/19490976.2021.1894070
49. Baker JM, Al-Nakkash L, Herbst-Kralovetz MM. Estrogen-gut microbiome axis: Physiological and clinical implications. Maturitas (2017) 103:45–53. doi: 10.1016/j.maturitas.2017.06.025
50. He S, Li H, Yu Z, Zhang F, Liang S, Liu H, et al. The gut microbiome and sex hormone-related diseases. Front Microbiol (2021) 12:711137. doi: 10.3389/fmicb.2021.711137
51. Kornman KS, Loesche WJ. Effects of estradiol and progesterone on bacteroides melaninogenicus and bacteroides gingivalis. Infect Immun (1982) 35:256–63. doi: 10.1128/iai.35.1.256-263.1982
52. Yurkovetskiy L, Burrows M, Khan AA, Graham L, Volchkov P, Becker L, et al. Gender bias in autoimmunity is influenced by microbiota. Immunity (2013) 39:400–12. doi: 10.1016/j.immuni.2013.08.013
53. Org E, Mehrabian M, Parks BW, Shipkova P, Liu X, Drake TA, et al. Sex differences and hormonal effects on gut microbiota composition in mice. Gut Microbes (2016) 7:313–22. doi: 10.1080/19490976.2016.1203502
54. Koren O, Goodrich JK, Cullender TC, Spor A, Laitinen K, Kling Bäckhed H, et al. Host remodeling of the gut microbiome and metabolic changes during pregnancy. Cell (2012) 150:470–80. doi: 10.1016/j.cell.2012.07.008
55. Mueller NT, Jacobs DR, MacLehose RF, Demerath EW, Kelly SP, Dreyfus JG, et al. Consumption of caffeinated and artificially sweetened soft drinks is associated with risk of early menarche. Am J Clin Nutr (2015) 102:648–54. doi: 10.3945/ajcn.114.100958
56. Mueller S, Saunier K, Hanisch C, Norin E, Alm L, Midtvedt T, et al. Differences in fecal microbiota in different European study populations in relation to age, gender, and country: A cross-sectional study. Appl Environ Microbiol (2006) 72:1027–33. doi: 10.1128/AEM.72.2.1027-1033.2006
57. Flores R, Shi J, Fuhrman B, Xu X, Veenstra TD, Gail MH, et al. Fecal microbial determinants of fecal and systemic estrogens and estrogen metabolites: A cross-sectional study. J Transl Med (2012) 10:253. doi: 10.1186/1479-5876-10-253
58. Chan JL, Kar S, Vanky E, Morin-Papunen L, Piltonen T, Puurunen J, et al. Racial and ethnic differences in the prevalence of metabolic syndrome and its components of metabolic syndrome in women with polycystic ovary syndrome: A regional cross-sectional study. Am J Obstet Gynecol (2017) 217:189.e1–8. doi: 10.1016/j.ajog.2017.04.007
59. Colldén H, Landin A, Wallenius V, Elebring E, Fändriks L, Nilsson ME, et al. The gut microbiota is a major regulator of androgen metabolism in intestinal contents. Am J Physiol Endocrinol Metab (2019) 317:E1182–92. doi: 10.1152/ajpendo.00338.2019
60. Fettweis JM, Serrano MG, Brooks JP, Edwards DJ, Girerd PH, Parikh HI, et al. The vaginal microbiome and preterm birth. Nat Med (2019) 25:1012–21. doi: 10.1038/s41591-019-0450-2
61. Kim YS, Kim N. Sex-gender differences in irritable bowel syndrome. J Neurogastroenterol Motil (2018) 24:544–58. doi: 10.5056/jnm18082
62. Ait-Belgnaoui A, Payard I, Rolland C, Harkat C, Braniste V, Théodorou V, et al. Bifidobacterium longum and lactobacillus helveticus synergistically suppress stress-related visceral hypersensitivity through hypothalamic-Pituitary-Adrenal axis modulation. J Neurogastroenterol Motil (2018) 24:138–46. doi: 10.5056/jnm16167
63. Distrutti E, Monaldi L, Ricci P, Fiorucci S. Gut microbiota role in irritable bowel syndrome: New therapeutic strategies. World J Gastroenterol (2016) 22:2219–41. doi: 10.3748/wjg.v22.i7.2219
64. Enck P, Azpiroz F, Boeckxstaens G, Elsenbruch S, Feinle-Bisset C, Holtmann G, et al. Functional dyspepsia. Nat Rev Dis Primers (2017) 3:17081. doi: 10.1038/nrdp.2017.81
65. Aytuğ N, Gi˙ral A, I˙meryüz N, Enç FY, Beki˙roğlu N, Aktaş G, et al. Gender influence on jejunal migrating motor complex. Am J Physiology-Gastrointest Liver Physiol (2001) 280:G255–63. doi: 10.1152/ajpgi.2001.280.2.G255
66. Hutson WR, Roehrkasse RL, Wald A. Influence of gender and menopause on gastric emptying and motility. Gastroenterology (1989) 96:11–7. doi: 10.1016/0016-5085(89)90758-0
67. Aloisi AM, Affaitati G, Ceccarelli I, Fiorenzani P, Lerza R, Rossi C, et al. Estradiol and testosterone differently affect visceral pain-related behavioural responses in Male and female rats. Eur J Pain (2010) 14:602–7. doi: 10.1016/j.ejpain.2009.10.016
68. Gunal O, Bozkurt A, Deniz M, Sungur M, Yeg BC. Effect of sex steroids on colonic distension-induced delay of gastric emptying in rats. J Gastroenterol Hepatol (2004) 19:975–81. doi: 10.1111/j.1440-1746.2004.03409.x
69. Browning KN, Travagli RA. Central nervous system control of gastrointestinal motility and secretion and modulation of gastrointestinal functions. In: Terjung R, editor. Comprehensive physiology. New York:Wiley (2014). p. 1339–68.
70. McEwen BS, Akama KT, Spencer-Segal JL, Milner TA, Waters EM. Estrogen effects on the brain: Actions beyond the hypothalamus via novel mechanisms. Behav Neurosci (2012) 126:4–16. doi: 10.1037/a0026708
71. Tache Y, Larauche M, Yuan P-Q, Million M. Brain and gut CRF signaling: Biological actions and role in the gastrointestinal tract. CMP (2018) 11(1):51–71. doi: 10.2174/1874467210666170224095741
72. Bradesi S, Eutamene H, Garcia-Villar R, Fioramonti J, Bueno L. Stress-induced visceral hypersensitivity in female rats is estrogen-dependent and involves tachykinin NK1 receptors. Pain (2003) 102:227–34. doi: 10.1016/S0304-3959(02)00056-8
73. Looijer-van Langen M, Hotte N, Dieleman LA, Albert E, Mulder C, Madsen KL. Estrogen receptor-β signaling modulates epithelial barrier function. Am J Physiology-Gastrointest Liver Physiol (2011) 300:G621–6. doi: 10.1152/ajpgi.00274.2010
74. Labus JS, Dinov ID, Jiang Z, Ashe-McNalley C, Zamanyan A, Shi Y, et al. Irritable bowel syndrome in female patients is associated with alterations in structural brain networks. Pain (2014) 155:137–49. doi: 10.1016/j.pain.2013.09.020
75. Cussotto S, Sandhu KV, Dinan TG, Cryan JF. The neuroendocrinology of the microbiota-Gut-Brain axis: A behavioural perspective. Front Neuroendocrinol (2018) 51:80–101. doi: 10.1016/j.yfrne.2018.04.002
76. Yuan X, Chen R, Zhang Y, Lin X, Yang X. Gut microbiota: Effect of pubertal status. BMC Microbiol (2020) 20:334. doi: 10.1186/s12866-020-02021-0
77. Shin J-H, Park Y-H, Sim M, Kim S-A, Joung H, Shin D-M. Serum level of sex steroid hormone is associated with diversity and profiles of human gut microbiome. Res Microbiol (2019) 170:192–201. doi: 10.1016/j.resmic.2019.03.003
78. Yuan X, Chen R, Zhang Y, Lin X, Yang X. Sexual dimorphism of gut microbiota at different pubertal status. Microb Cell Fact (2020) 19:152. doi: 10.1186/s12934-020-01412-2
79. Korpela K, Kallio S, Salonen A, Hero M, Kukkonen AK, Miettinen PJ, et al. Gut microbiota develop towards an adult profile in a sex-specific manner during puberty. Sci Rep (2021) 11:23297. doi: 10.1038/s41598-021-02375-z
80. Ringel-Kulka T, Cheng J, Ringel Y, Salojärvi J, Carroll I, Palva A, et al. Intestinal microbiota in healthy U.S. young children and adults–a high throughput microarray analysis. PloS One (2013) 8:e64315. doi: 10.1371/journal.pone.0064315
81. Hollister EB, Riehle K, Luna RA, Weidler EM, Rubio-Gonzales M, Mistretta T-A, et al. Structure and function of the healthy pre-adolescent pediatric gut microbiome. Microbiome (2015) 3:36. doi: 10.1186/s40168-015-0101-x
82. Del Chierico F, Abbatini F, Russo A, Quagliariello A, Reddel S, Capoccia D, et al. Gut microbiota markers in obese adolescent and adult patients: Age-dependent differential patterns. Front Microbiol (2018) 9:1210. doi: 10.3389/fmicb.2018.01210
83. Lin HV, Frassetto A, Kowalik EJ Jr., Nawrocki AR, Lu MM, Kosinski JR, et al. Butyrate and propionate protect against diet-induced obesity and regulate gut hormones via free fatty acid receptor 3-independent mechanisms. PloS One (2012) 7:e35240. doi: 10.1371/journal.pone.0035240
84. Fuhrman BJ, Feigelson HS, Flores R, Gail MH, Xu X, Ravel J, et al. Associations of the fecal microbiome with urinary estrogens and estrogen metabolites in postmenopausal women. J Clin Endocrinol Metab (2014) 99:4632–40. doi: 10.1210/jc.2014-2222
85. Evans JM, Morris LS, Marchesi JR. The gut microbiome: The role of a virtual organ in the endocrinology of the host. J Endocrinol (2013) 218:R37–47. doi: 10.1530/JOE-13-0131
86. Kimura I, Ichimura A, Ohue-Kitano R, Igarashi M. Free fatty acid receptors in health and disease. Physiol Rev (2020) 100:171–210. doi: 10.1152/physrev.00041.2018
87. Dong G, Zhang J, Yang Z, Feng X, Li J, Li D, et al. The association of gut microbiota with idiopathic central precocious puberty in girls. Front Endocrinol (Lausanne) (2019) 10:941. doi: 10.3389/fendo.2019.00941
88. Delzenne NM, Cani PD. Interaction between obesity and the gut microbiota: Relevance in nutrition. Annu Rev Nutr (2011) 31:15–31. doi: 10.1146/annurev-nutr-072610-145146
89. Chakraborti CK. New-found link between microbiota and obesity. WJGP (2015) 6:110. doi: 10.4291/wjgp.v6.i4.110
90. Neuman H, Debelius JW, Knight R, Koren O. Microbial endocrinology: The interplay between the microbiota and the endocrine system. FEMS Microbiol Rev (2015) 39:509–21. doi: 10.1093/femsre/fuu010
91. Matkovic V. Leptin is inversely related to age at menarche in human females. J Clin Endocrinol Metab (1997) 82:3239–45. doi: 10.1210/jc.82.10.3239
92. Rutters F, Nieuwenhuizen AG, Verhoef SPM, Lemmens SGT, Vogels N, Westerterp-Plantenga MS. The relationship between leptin, gonadotropic hormones, and body composition during puberty in a Dutch children cohort. Eur J Endocrinol (2009) 160:973–8. doi: 10.1530/EJE-08-0762
93. Li Y, Shen L, Huang C, Li X, Chen J, Li SC, et al. Altered nitric oxide induced by gut microbiota reveals the connection between central precocious puberty and obesity. Clin Transl Med (2021) 11:e299. doi: 10.1002/ctm2.299
Keywords: precocious puberty (PP), gut, microbioma, sex hormone, axis
Citation: Calcaterra V, Rossi V, Massini G, Regalbuto C, Hruby C, Panelli S, Bandi C and Zuccotti G (2022) Precocious puberty and microbiota: The role of the sex hormone–gut microbiome axis. Front. Endocrinol. 13:1000919. doi: 10.3389/fendo.2022.1000919
Received: 22 July 2022; Accepted: 05 October 2022;
Published: 21 October 2022.
Edited by:
Madhusmita Misra, Massachusetts General Hospital and Harvard Medical School, United StatesReviewed by:
Ahreum Kwon, Yonsei University, South KoreaHavva Nur Kendirci, Hittite University, Turkey
Copyright © 2022 Calcaterra, Rossi, Massini, Regalbuto, Hruby, Panelli, Bandi and Zuccotti. This is an open-access article distributed under the terms of the Creative Commons Attribution License (CC BY). The use, distribution or reproduction in other forums is permitted, provided the original author(s) and the copyright owner(s) are credited and that the original publication in this journal is cited, in accordance with accepted academic practice. No use, distribution or reproduction is permitted which does not comply with these terms.
*Correspondence: Valeria Calcaterra, valeria.calcaterra@unipv.it