Assessing Amino Acid Metabolism in Splanchnic Tissues and Mammary Glands to Short-Term Graded Removal of Lys From an Abomasal-Infused Amino Acid Mixture in Lactating Goats
- Ruminant Nutrition and Physiology Laboratory, College of Animal Science and Technology, Shandong Agricultural University, Taian, China
To investigate the responses of amino acid metabolism in portal-drained viscera (PDV), liver, and mammary glands (MGs) to a graded gradual decrease of post-ruminal Lys supply, four multi-catheterized lactating goats were used in a 4 × 4 Latin square experiment. Goats were fasted for 12 h and then received a 33-h abomasal infusion of an amino acid mixture and glucose. Treatments consisted of a graded decrease of Lys content in the infusate to 100 (complete), 60, 30, or 0% as in casein. Lys-removed infusions decreased the production of milk, milk protein, fat, and lactose linearly and also decreased arterial Lys concentrations linearly (p < 0.05). Net PDV uptake decreased linearly (p < 0.05) with decreasing PDV loss ratio (p < 0.05). Although liver removal of Lys decreased linearly (p < 0.05), the removal ratio relative to portal absorption changed small, which was about 10% in all four treatments. Reduced Lys supply resulted in a linear decrease in the utilization of Lys in the peripheral tissues (except mammary, p < 0.05) and the release of more Lys in MGs. Although net mammary uptake of Lys declined linearly (p < 0.05), lactating goats can partially offset the negative effect of decreased circulating Lys concentrations by increasing mammary affinity (p <0.05) and increasing mammary blood flow (p < 0.05). Graded removal of Lys from the infusate linearly decreased mammary uptake-to-output ratios of Lys (p < 0.05) suggesting that mammary catabolism of Lys decreased. Meanwhile, the treatments linearly increased circulating concentrations of glucagon and linearly decreased prolactin (p < 0.05). In conclusion, the results of the present study indicated that there were several mechanisms used to mitigate a Lys deficiency, including reduced catabolism of Lys in PDV and peripheral tissues (including MGs) and linearly increased mammary blood flow and mammary affinity together with increased mammary uptake and U:O of branched-chain amino acids (BCAA). Given these changes, the decline in milk protein production could be attributed to the combined effect of mass action with Lys and hormonal status.
Introduction
Lactating ruminants usually have lower efficiency of nitrogen (N) utilization than growing animals, which result in a big environmental problem, such as soil and water eutrophication of N (1, 2). Postabsorptive efficiency of N use for milk is determined by the amount of metabolizable protein (MP) supplied relative to animal needs, the profile of absorbed EAA, and the rate of protein synthesis based on the present knowledge of amino acid (AA) nutrition (3), so improving the postabsorptive N efficiency without sacrificing milk protein production is a major strategy for increasing overall dietary N efficiency.
The losses of absorbed AA mainly occur in the portal-drained viscera (PDV), liver, peripheral tissues, and metabolic pathways other than only milk protein synthesis in the mammary glands (MGs) (4). In adult lactating cows, the net AA consumption from the PDV, liver, and MG was roughly equal to absorption, while the loss of AA metabolism in other tissues was low (5). Therefore, predicting changes in the rate and extent of AA metabolism by the PDV, liver, and MGs is an essential prerequisite for improving the precision of models that predict the MP requirements of dairy ruminants (6, 7).
Total AA losses during absorption are proportional to MP supply (4), but there is a variation in gut-associated losses among individual AA (8) that the metabolic disappearance rate of each EAA flowing through PDV ranges from 10.3 to 45.8% (7). Analysis of related research results shows that the liver catabolizes about 45% of the total AA flowing into the portal vein (4), but for individual EAA, the catabolized ratio varies widely, ranging from −0.4 to 55.2% (7). Changes in plasma AA profiles in response to casein infusion indicate that His, Met, and Phe plus Tyr are removed by the liver in substantial proportions of their net portal appearance, whereas Ile, Leu, Lys, and Val are barely extracted by the liver (9). Therefore, the relationship between individual EAA supply and metabolic loss in PDV and liver needs further investigation.
The mammary uptake to milk output ratio (U:O) of total amino acids is 96%−105%, indicating that the catabolism of amino acids does not occur under basically normal physiological conditions; the mammary uptake to milk output ratio of EAA and nonessential amino acids (NEAA) is 66%−79% and 141%−182%, respectively, indicating that part of EAA is converted to NEAA in the MGs (10). In general, Lys and branched-chain amino acids (BCAA) are taken up in excess relative to milk output, whereas Met, His, Phe, and Trp are taken up in approximately the same amount as in milk protein secretion (11, 12). Due to the EAA supply balance regulation mechanism of EAA supply at tissue and cell levels, in a certain range, the change of a single EAA supply may not lead to a change in milk protein production, but it will affect the lactational efficiency of the EAA.
Lys is often the limiting AA for milk protein synthesis in lactating ruminants. Increasing Lys in MP to more optimal concentrations by post-ruminal infusion (13, 14) or by feeding a rumen-protected Lys supplement (15, 16) increased milk protein yield.
Nitrogen originating from Lys appeared in Arg, Asp, Ala, Glu, and Ser concomitant with excess mammary uptake of Lys in dairy cows (17). As the deletion of Lys from an AA mixture decreased milk protein yield (18), this might suggest the excess uptake of Lys across the MGs may be obligate to maintain milk protein production by supporting NEAA synthesis.
Starvation-refeeding experiments, in which animals receive nutrients per os or by infusion after being deprived for 12–48 h, have been very useful in illustrating the postprandial response of dietary components (19, 20). It has been widely used in lactating rats, goats, and cows (21–24). The objective of this study was to determine how the PDV, liver, and MGs respond to varying short-term supplies of Lys in catheterized dairy goats. As Lys supply varies, splanchnic tissues and MGs are hypothesized to actively regulate AA catabolism.
Materials and Methods
Animals and Diet
Four multiparous Laoshan dairy goats averaging 60 ± 10 day in milk (DIM) and 50 ± 5 kg of body weight (BW) were used at the beginning of the experiment. Surgeries were performed at least 1 month before the start of the experiment in which all goats were surgically implanted with medical silicone catheters (1 mm × 2 mm, Chensheng Medical, China) in the portal vein, hepatic vein, and one mesenteric vein (25). A T-type Tygon (8 mm × 10 mm, Weili, China) was surgically implanted in the abomasum, and the right carotid artery was also raised to a subcutaneous position to provide access to arterial blood. The diet was formulated according to Agricultural and Food Research Council (AFRC) (26), which could provide 10.65 MJ ME and 110.7 g MP/kg DM (Table 1). The daily amount of diet offered was adjusted to maintain a 5% refusal rate. Goats had ad libitum access to water throughout the experiment.
Experimental Design and Procedure
The experimental design was a randomized 4 × 4 Latin square with four 14-day periods. Treatments consisted of abomasal infusion of an AA mixture containing 100 (complete), 60, 30, or 0% of the Lys present in casein. The profile of casein was similar in composition to bovine milk casein in Table 2 (27). Lys was substituted by equal moles of Glu to achieve Lys-deficient infusates and, because of solubility limitations, all the Tyr was replaced by Phe. The infusion rate was set to match the MP requirement of each goat according to AFRC (26) based on milk yield recorded for 3 consecutive days before the start of infusion in each period. The amount of glucose infused was calculated to provide 3.6 mg glucose/kg BW per minute according to the observed metabolic use rate measured in lactating goats by using isotopic tracer technology (28). In each experimental period, goats were moved to individual metabolic cages and fasted for 12 h before the start of the infusion to mitigate possible interference from absorbed Lys from the remaining digesta. The infusion began at 08:00 on the 1st day and lasted 33 h to ensure steady blood glucose and urea concentration, which need about 15 h for glucose and 20 h for urea to establish according to the result of our pre-experiment (Figure 1). Samples were collected on the 2nd day. After stopping the infusion, goats were allowed for a 12-day rest in individual free stalls before the start of the next period.
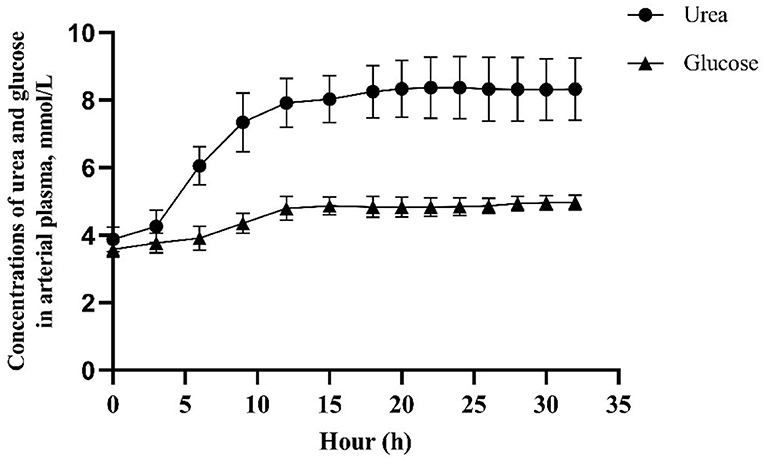
Figure 1. Concentrations of urea and glucose in arterial plasma after infusion of glucose and amino acid into abomasum in a preliminary experiment.
The AA mixture and glucose were dissolved in 1,920 ml of saline each day, which was adjusted to a pH of 7.4 using HCl and NaOH and was infused continuously through a peristaltic pump (Type H-L2, Huxi Analytical Instrument Factory, Shanghai, China) to abomasum at a rate of 80 ml/h for the duration of the study. During the resting periods, goats were milked two times daily at 08:00 and 18:00 and allowed free access to the pelleted diet and water.
Sampling and Analysis
Samples were collected on the 2nd day during each infusion period. Milk samples were collected between 08:00 and 17:00, which was weighed, recorded, and sampled for later analysis. Milk was divided into two subsamples: one was used for routine analysis using an infrared milk composition analyzer (Type 76110, FOSS, Denmark) and the other for AA determination after hydrolysis using an AA analyzer (Type L-8900, Hitch, Japan). Samples for AA analysis were sent to be hydrolyzed with hydrochloric acid at a final concentration of 6 M. The hydrolysate was filtrated through a 0.2-μm film before being loaded into the AA analyzer. To determine plasma flow across the splanchnic tissues, para-amino hippuric acid (pAH; 0.48g/h, 1% wt/vol) was continuously infused into the mesenteric vein catheter using a syringe pump (Type 50F6, SN, China) from 10:00 to 16:00 h. The pAH was infused 1.5 h before the first blood sample was collected, followed by a priming dose (0.2 g) for 5 min. On each sampling day, four sets of arterial, portal venous, and hepatic venous blood samples were collected simultaneously at 90-min intervals beginning at 11:30. Mammary venous blood samples were taken by venipuncture immediately after the withdrawal of the splanchnic blood samples. Blood samples were also divided into two samples, one was placed on ice and centrifuged (10 min, 1,800 × g at 4°C) within 30 min of collection to yield plasma, and the other one was for the packed cell volume by the automatic analyzer of blood cell (Mindray, BC-6100Plus). A total of four samples were pooled together and kept at −80°C for later analysis of free AA concentration using an AA analyzer (Type L-8900, Hitch, Japan). Samples were deproteinized by mixing with equal amounts of 10% sulfosalicylic acid, kept at 4°C overnight, and centrifuged at 15,000 × g for 20 min. The extracted supernatant was passed through a 0.2-μm film before being loaded into the AA analyzer. Arterial plasma was subsampled for total protein, albumin, urea-N, and glucose analysis by an automatic biochemical analyzer (Type 7020, Hitch, Japan). Hormones such as insulin, glucagon, and prolactin were determined by radioimmunoassay using commercial assay kits (Tianjin Xiehe Medical Technology Co. Ltd.).
Calculations and Statistical Procedures
Blood and plasma flow were calculated from the downstream dilution of pAH infused into mesenteric catheters (29). Daily averages of blood flows were used to calculate the net fluxes of AA. Mammary plasma flow was estimated according to the Fick principle using Phe and Tyr as internal markers (30). The net fluxes of AA across the PDV, liver, total splanchnic tissues (TSP), and MG were calculated for each goat period as the product of the average plasma venous–arterial concentration difference and the average blood flow. A negative flux indicates utilization or removal, whereas a positive flux indicates net production or release of the nutrient across the tissue (31). Mammary uptake to milk output ratios (U:O) for individual AA were calculated as the mammary uptake of AA divided by the amount secreted in milk during the 9 h between milkings. PDV clearance rates (Kp), liver clearance rates (Kh), and mammary clearance rates (Km) were calculated to assess tissue affinity for blood metabolites (32):
where Fa, Fp, Fh, and Fm refer to arterial, portal venous, hepatic venous, and mammary blood flows, respectively (L/h); Ca, Cp, Ch, and Cm refer to arterial venous, portal venous, hepatic venous, and mammary venous AA concentrations (μmol/L).
Data were subjected to mixed procedure analysis by SAS (version 9.2, SAS Institute, Inc., Cary, NC, USA). The statistic model used was
where Y was the observed value for the kn treatment, the jn period, and in goat; μ was the overall mean; Gi was the random effect of goat, Pj was the random effect of the period, Tk was the fixed effect of Lys dose effects, and eijk was the random error associated with Yijk. Both linear and quadratic effects of Lys dose were initially tested. When quadratic effects were nonsignificant, they were removed from the model. The results were expressed as least square means (LSM) with standard error of the mean (SEM). Significance was declared at p ≤ 0.05 and tendency was declared at p ≤ 0.1. Multiple comparisons between treatment means were made using the Tukey method.
Results
Milk Yields and Composition
Removal of Lys from the infused mixture decreased the production of milk, milk protein, milk fat, milk lactose, and the content of milk protein linearly (p < 0.05, Table 3). Production of milk, milk protein, milk fat, and milk lactose in the 100 and 60% treatments were greater than in the 30 and 0% treatments. The content of milk protein in the 100 and 60% treatments were higher than the 0% treatment.
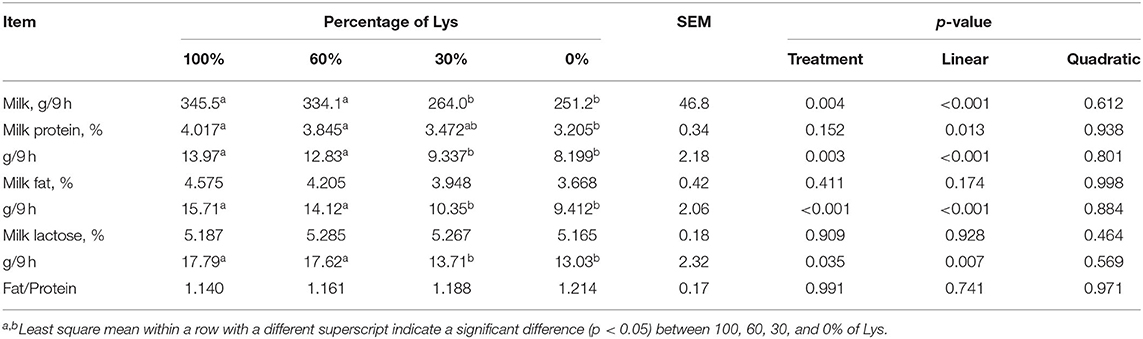
Table 3. Effects of graded removal of Lys from the amino acid mixture infused into abomasum on lactation performance of lactating goats.
Arterial-Free AA
Lys removal decreased arterial Lys concentration linearly (p < 0.05, Table 4). Removing all Lys from the infusate decreased arterial Lys to about one-fifth of the full mixture infusion. Arterial concentrations of all the other measured AA, BCAA, EAA, NEAA, and TAA were unaffected by Lys removal from the infusions.
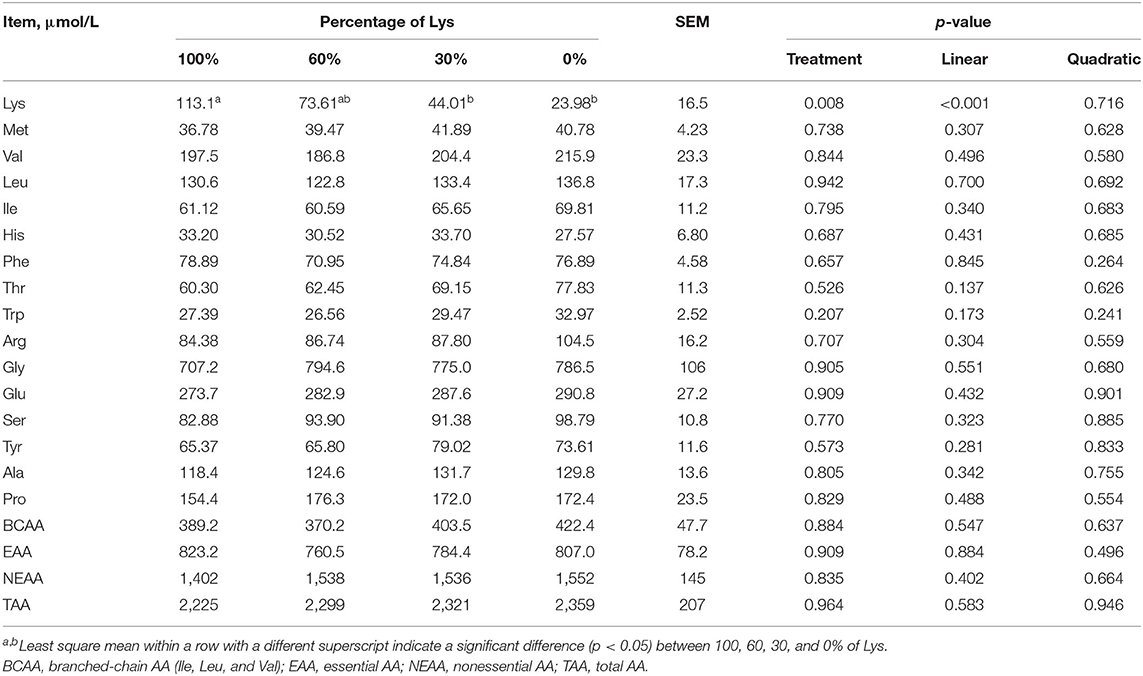
Table 4. Effects of graded removal of Lys from the amino acid mixture infused into abomasum on amino acid concentrations in the plasma of carotid artery in lactating goats.
Arterial Metabolites, Hormones, and Blood Flow
Arterial concentrations of all measured metabolites and hormones were unaffected, apart from glucagon, which increased linearly, and prolactin, which decreased linearly by Lys removal from the infusions (p < 0.05, Table 5). Glucagon in the 0% treatment was greater than the 100% treatment and the prolactin in the 100% treatment was greater than the other three treatments. Graded removal of Lys from the infusate linearly increased mammary blood flow (p < 0.05, Table 6) than the 0% group which was greater than the 100% group. Portal and splanchnic blood flow were unaffected by Lys removal from the infusions.
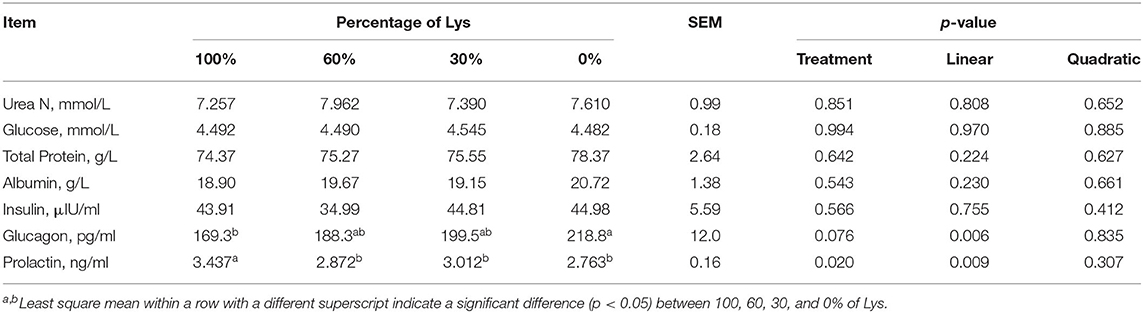
Table 5. Effects of graded removal of Lys from the AA mixture infused into abomasum on concentrations of metabolites and hormones in the plasma of carotid artery in lactating goats.

Table 6. Effects of graded removal of Lys from the AA mixture infused into abomasum on splanchnic blood flows in lactating goats.
Net Fluxes of Individual AA Across Tissues
The linear decrease in the Lys infusion rate was accompanied by a linear increase in the Glu infusion rate because the missing Lys was replaced by an equal mole of Glu. Net fluxes of individual AA across the PDV, heptic tissues (HEP), TSP, MG, and in milk are presented in Table 7. Graded removal of Lys from the infusate linearly decreased the net fluxes of PDV, HEP, TSP, MG, and milk (p < 0.05). Removing all Lys from the infusate decreased the net fluxes of PDV and TSP to about one-sixth of the full mixture infusion. Upon removal of the liver, the amounts of Lys decreased about 85% from 100 to 0% treatment. Mammary uptake of Lys decreased sharply when the infusion of Lys decreased from 60 to 30%. The hepatic removal of Arg was linearly affected by the graded removal of Lys (p < 0.05). Net fluxes across the TSP of Leu tended to decrease linearly with the graded removal of Lys (0.05 < p < 0.1). Across the MG, the graded removal of Lys caused a linear increase in the uptake of Ile and Val (p < 0.05). All the other net fluxes of AA were unaffected by Lys removal from the infusions.
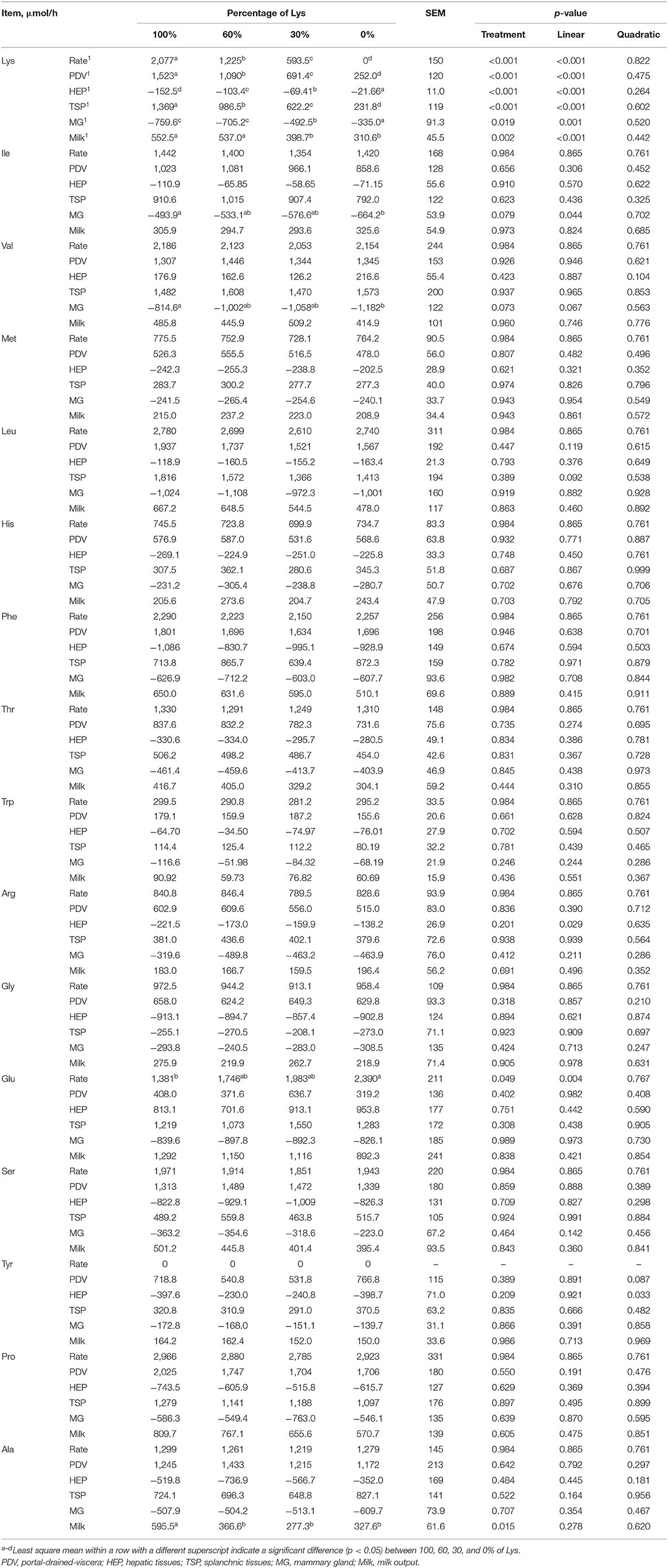
Table 7. Effects of graded removal of Lys from the AA mixture infused into abomasum on splanchnic and mammary net fluxes of amino acids in lactating goats.
PDV, Liver, and Mammary Clearance Rates of AA
The PDV clearance rate of Lys and Val decreased linearly with the graded removal of Lys (p < 0.05, Table 8). Numerically, the PDV clearance rate of Lys decreased by 2.43-folds for the 30% Lys treatment compared with that of complete mixture infusion. All the other measured EAA were unaffected with graded removal of Lys.
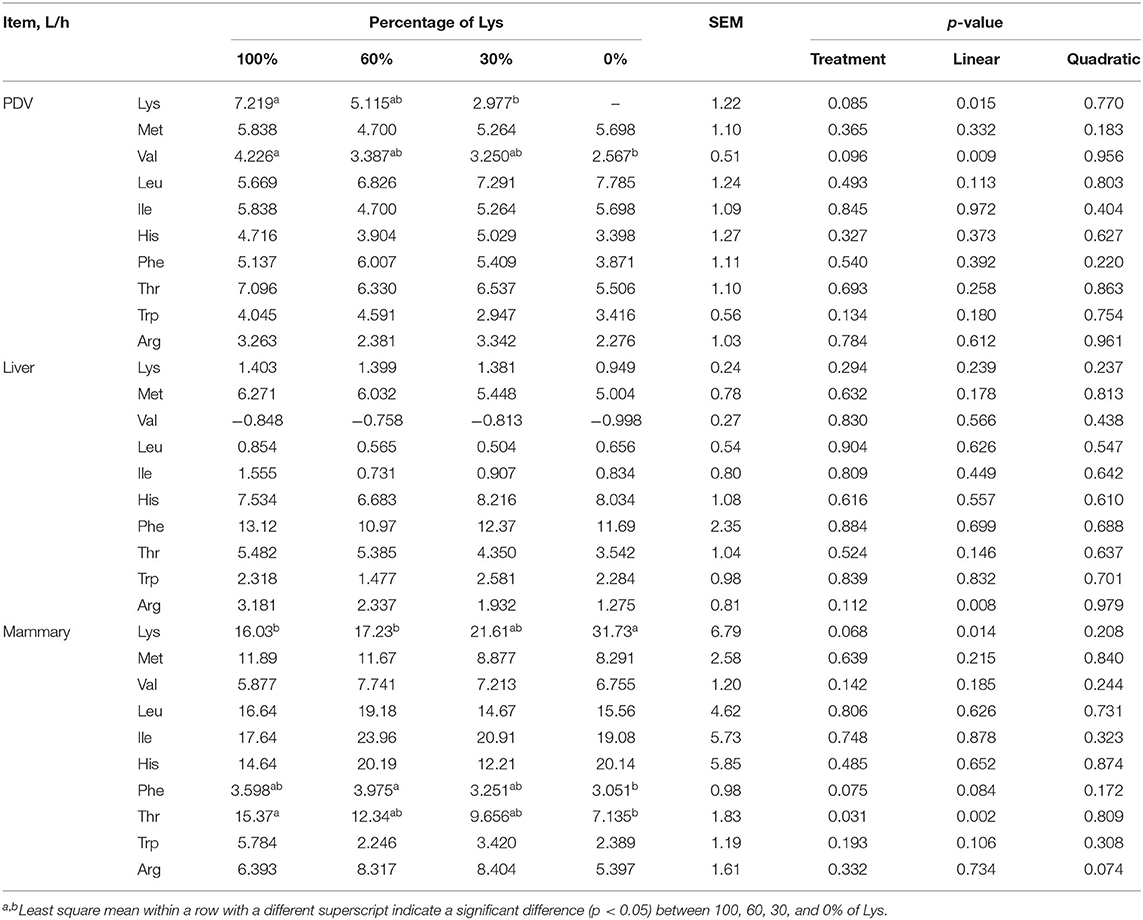
Table 8. Effects of graded removal of Lys from the AA mixture infused into abomasum on PDV, liver and mammary amino acids clearance rates of lactating goats.
The liver clearance rate of Arg decreased linearly (p < 0.05). Graded removal of Lys from the infusate had no significant effect on liver clearance rates of the other EAA measured.
Graded removal of Lys from the infusate increased the mammary clearance rate of Lys linearly (p < 0.05). Numerically, the mammary clearance rate of Lys increased by 2.06-folds for the 0% Lys treatment compared with that of complete mixture infusion. The mammary clearance rate of Thr decreased linearly (p < 0.05) while mammary clearance for Phe tended to decrease linearly (0.05 < p < 0.1) with decreasing the infusion of Lys.
U:O of AA
Graded removal of Lys from the infusate decreased linearly mammary U:O of Lys to a significant level (p < 0.05; Table 9). The mammary U:O of Lys in the 100% treatment was greater than 30% and 0% treatments. Graded removal of Lys from the infusate linearly increased the mammary U:O of Leu and Ile. Treatments affected the U:O of Arg quadratically (p < 0.05). U:O increased and then decreased with decreasing Lys content in the infusate. Treatments had no significant effects on mammary U:O of the other AA measured.
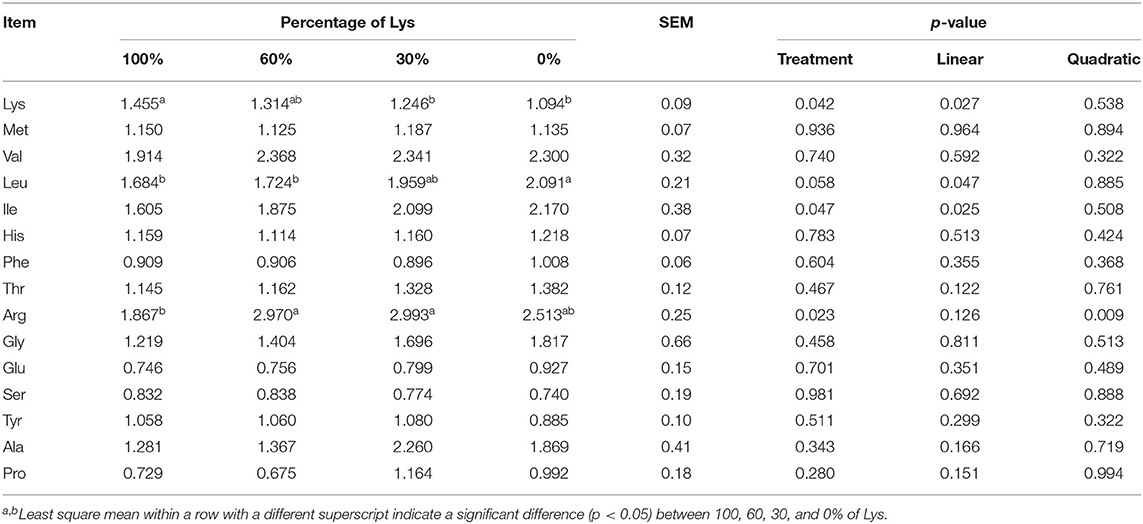
Table 9. Effects of graded removal of Lys from the AA mixture infused into abomasum on the ratio of mammary uptake to milk output in lactating goats.
Discussion
Milk Protein Production
As indicated in previous studies, the extent of Lys deficiency in the experiment was limited by the Lys supply from the basal diet, and there was little chance of having a significant change in milk protein production. For example, of the seven experiments that investigated the response of milk protein to varied post-ruminal Lys supply since 2000, six experiments observed no significant effects (16, 33–37), and the other reported lower milk protein production for the low MP diet supplemented with Lys compared with that of the MP-adequate diet (38). Therefore, such experimental procedures are inappropriate if we only want to investigate the effects of individual EAA on milk protein synthesis. To avoid the limitation of basal diets on the extent of individual AA deficiency, one experiment was designed by subtracting approximately 45% of each EAA from the full mixture of EAA infused into the cows. This experiment was to investigate the effect of the post-ruminal supply of individual EAA on milk protein production and found that the removal of Lys caused the greatest depression in milk protein production (39). Another experiment found that graded removal of Lys from an intravenously infused amino acid mixture linearly decreased milk protein production (3). In this study, graded removal of Lys from the infusate also linearly decreased milk protein production. Considering the concomitant linear decrease in mammary Lys uptake (Table 7), it seemed reasonable to interpret the result as a mass action of Lys supply. Meanwhile, we observed a linear increase in circulating glucagon and a decrease in circulating prolactin by a graded removal of Lys from the infusate in the present study. It has been observed that the infusion of glucagon decreased milk protein production in lactating cows (40, 41). Prolactin also plays an important role in mammary development and lactation (42). Inhibition of the release of prolactin significantly reduced milk protein yield in dairy goats and cows (43–46). Thus, Lys supply may exert a portion of its effects on milk protein synthesis through glucagon and prolactin. As mentioned above, a variation in post-ruminal Lys supply can affect milk protein synthesis through mass action with Lys and a change in hormonal status.
Splanchnic and Mammary Net Fluxes of Amino Acids
As revealed by previous studies, decreasing the post-ruminal supply of an EAA would generally decrease the circulating concentration of that specific EAA (3, 36, 47). Consistent with these expectations, arterial concentrations of Lys decreased linearly with the removal of Lys in the present study. Compared with the complete mixture of the 100% treatment, the removal of all Lys reduced arterial Lys concentrations to 21%. Arterial concentrations of other EAAs were not affected by treatment, indicating that the metabolism of individual EAA usually had an independent effect on protein synthesis (48).
The splanchnic and mammary net flux of AA is closely related to blood flow. The contribution of portal blood flow to splanchnic blood flow ranges from 75 to 85% (49–51). In this study, portal blood flow (95.7 L/h) was 78% of splanchnic blood flow (122.7 L/h), which was consistent with previous studies of lactating ruminants. The splanchnic blood flow regulated by energy supply rather than protein supply (52) had no response to graded removal of Lys. Graded removal of Lys linearly increased mammary blood flow. This phenomenon was observed for Met removal (24), Lys removal (3), His deficiency (53), and Leu deficiency (27). Therefore, increased mammary blood flow appears to be a common response in response to deficiencies of individual EAA (54), which helps to mitigate the deficiency. NO concentration in the mammary vein plasma was linearly elevated by Met removal (24) and Lys removal (3) in the previous experiments. This may at least partially explain the elevated mammary blood flow as NO has been identified as one of the local vasodilatory compounds (55). The mechanisms of AA that induced the increased MG blood flow need further investigation.
Amino acid metabolism by the PDV tissue affects the net supply and composition of AA entering the bloodstream (4). Gut use of AA also varies by different AAs with the loss of His being very small, while the loss of Leu, Thr, and some NEAA was much greater (56). The same phenomenon occurred in this experiment, where the net recovery ratio of His was greatest at about 80%, but the BCAA only averaged about 63%. If the PDV flux of the 0% Lys treatment was taken as the basal absorption, the metabolic loss of Lys in the PDV is 39.2, 31.6, and 26.3% for 100, 60, and 30% treatments, respectively. Meanwhile, the PDV clearance rates for Lys decreased linearly from 7.219 to 2.977 L/h. Thus, decreases in post-ruminal Lys supply resulted in decreased utilization by the digestive tract and increased relative availability of Lys for other tissues due to decreased affinity of Lys in PDV. PDV flux of other AAs was not significantly affected, indicating that the metabolic loss rate of PDV can be altered by adjusting an EAA supply.
If all AAs are considered together, then the liver removes an average of about 45% of portal absorption (4). However, this value hides the considerable variation in how the individual AA is handled and the impact this has on post-hepatic supply and metabolism. In this experiment, the net removal of Met, His, Phe, Thr, Trp, and Arg by the liver was relatively large, between 35 and 60%, while the removal of Lys and BCAA by the liver was very small. Although the net liver flux of Lys decreased linearly, the removal ratio of portal absorption changed small, which was about 10% at the four treatments. At the same time, the clearance rate of Lys by the liver also showed a nonsignificant change with decreasing Lys supply (Table 8). This suggests that the liver can remove Lys in a fixed proportion in lactating ruminants.
Post-liver supply was greater than mammary uptake, which exceeded milk output, as is usually observed for Lys (4). The gradual removal of Lys resulted in a linear decrease in the amount of Lys delivered to peripheral tissue (net TSP flux) and mammary uptake (Table 8) in this experiment, which was similar to a previous study (34). The mammary uptake amount of Lys accounts for more and more peripheral tissue supply with decreasing post-ruminal Lys supply, indicating that the utilization of Lys in peripheral tissues (non-mammary) is reduced. Mammary uptake and milk output of Lys was even higher than the post-liver supply in the 0% treatment, this may be due to the mobilization of body protein reserves to meet the requirement of lactation (51). There were no significant changes in the mammary uptake of Lys between 100 and 60% treatments despite large declines in arterial Lys concentrations of about 35% between 100 and 60% treatments. Changes in mammary blood flow and mammary affinity compensated for part of the varied supply of individual EAA, thereby mitigating the responses (3, 53). However, there was a limit to this buffering because the mammary uptake of Lys decreased significantly when the post-ruminal supply of Lys was reduced to 30%.
Branched-chain amino acids and Lys are taken up in excess by the MGs (57). It is known that Lys can be used to support necessary NEAA synthesis in MGs (17) and its utilization in mammary is sensitive to nutrient supply (58). The mammary U:O of Lys decreased linearly from 1.45 to 1.09, which is similar to a previous study that U:O decreased when decreasing doses of Lys supply (34, 57, 59). Although MGs could decrease U:O, the values always exceeded unity, even at 0% treatment. This suggested that there are other roles for Lys within the MG, such as supporting necessary NEAA synthesis (17). Meanwhile, increased mammary uptake of Ile and Val and increased U:O of Leu and Ile compensated for the lowered metabolism of Lys because BCAA could provide N for NEAA synthesis (34).
Mechanisms to Mitigate the Lys Deficiency
The main innovation of this study was that we can observe the route of AA absorbed into milk protein and the response of amino acid metabolism in the PDV, liver, and MG to the change of Lys supply by using the multi-catheterized model of dairy goats, this provides a new strategy to improve postabsorptive N efficiency. The infusion rate of Lys decreased by about 2,100 μmol/h from 100 to 0% treatment. Nonetheless, mammary uptake only decreased by 424 μmol/h while Lys in milk protein secretion decreased only by about 242 μmol/h from 100 to 0% treatment. According to the outcomes of this experiment, there are several pathways to mitigate Lys deficiency in lactating ruminants. First, lactating ruminants adapted to Lys deficiency by reducing net utilization or catabolism in PDV and peripheral tissues (except mammary, Figure 2). There may be a limit to this adaptation; however, as mammary uptake was still impacted negatively when the post-ruminal supply of Lys decreased to 30% and 0%. Second, lactating ruminants enhanced mammary uptake ability to Lys due to increased mammary blood flow and mammary affinity (Tables 6, 8). Lastly, the utilization or catabolism of Lys in the MG is reduced along with the increased mammary uptake and U:O of BCAA to compensate for the lowered metabolism of Lys. From all of the above, we can include that lactating ruminants have a priority to meet the requirement of lactation.
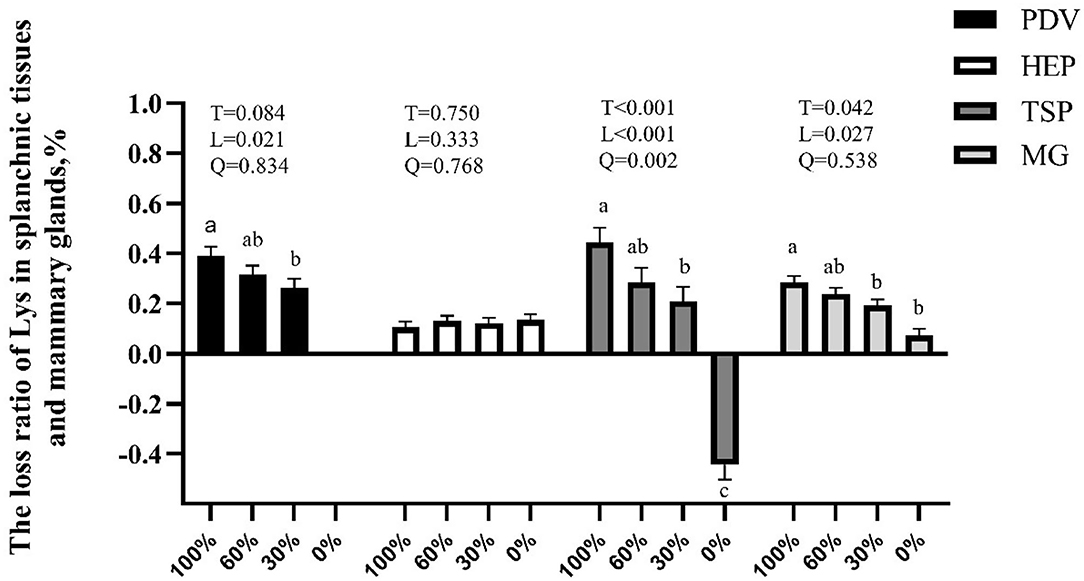
Figure 2. Effects of graded removal of Lys from the amino acid (AA) mixture infused into abomasum on the loss ratio of Lys in splanchnic tissues and mammary glands of lactating goats. PDV, portal-drained-viscera; HEP, hepatic tissues; TSP, splanchnic tissues; MG, mammary gland. a–cLeast square mean within a row with a different superscript indicate a significant difference (p < 0.05) between 100, 60, 30, and 0% of Lys.
Conclusion
Lactating ruminants use several mechanisms to mitigate Lys deficiencies, including reduced utilization of Lys by PDV and peripheral tissues (including MGs), linear increases in mammary blood flow, and mammary affinity for Lys. This can include the lactating ruminants having a priority to meet the requirement of lactation. Graded removal of Lys from the abomasum-infused AA mixture linearly decreased milk protein production. The decrease was a combinative result of mass action with Lys and changed hormonal status with increased glucagon and decreased prolactin. Further work is required to delineate external hormonal effects and intracellular cell signaling effects.
Data Availability Statement
The original contributions presented in the study are included in the article/supplementary material, further inquiries can be directed to the corresponding author.
Ethics Statement
The animal study was reviewed and approved by Institutional Animal Care and Use Committee of Shandong Agricultural University.
Author Contributions
YL and ZW: conceptualization and methodology. YL and CL: formal analysis. YL: writing the original draft preparation. XL, ZH, QH, and ZW: writing—review and editing. XL and ZW: project administration and supervision. All authors contributed to the article and approved the submitted version.
Funding
This work was funded by the National Natural Science Foundation of China under Project No. 31772623 and by the China Agriculture Research System of MOF and MARA.
Conflict of Interest
The authors declare that the research was conducted in the absence of any commercial or financial relationships that could be construed as a potential conflict of interest.
Publisher's Note
All claims expressed in this article are solely those of the authors and do not necessarily represent those of their affiliated organizations, or those of the publisher, the editors and the reviewers. Any product that may be evaluated in this article, or claim that may be made by its manufacturer, is not guaranteed or endorsed by the publisher.
References
1. Howarth RW, Boyer EW, Pabich WJ, Galloway JN. Nitrogen use in the United States from 1961–2000 and potential future trends. Ambio. (2002) 31:88–96. doi: 10.1579/0044-7447-31.2.88
2. Haro A, Gonzalez J, Evan TD, Fuente J, Carro M. Effects of feeding rumen-protected sunflower seed and meal protein on feed intake, diet digestibility, ruminal, cecal fermentation, and growth performance of lambs. Animals. (2019) 9:415–25. doi: 10.3390/ani9070415
3. Guo CL Li YT, Lin XY, Hanigan MD, Yan ZG, Hu ZY, et al. Effects of graded removal of lysine from an intravenously infused amino acid mixture on lactation performance and mammary amino acid metabolism in lactating goats. J Dairy Sci. (2017) 100:4552–64. doi: 10.3168/jds.2016-11921
4. Lapierre H, Berthiaume R, Raggio G, Thivierge MC, Doepel L, Pacheco D, et al. The route of absorbed nitrogen into milk protein. Anim Sci. (2005) 80:11–22. doi: 10.1079/ASC41330011
5. Larsen M, Galindo C, Ouellet DR, Maxin G, Kristensen NB, Lapierre H. Abomasal amino acid infusion in postpartum dairy cows: effect on whole-body, splanchnic, and mammary amino acid metabolism. J Dairy Sci. (2015) 98:7944–61. doi: 10.3168/jds.2015-9439
6. Hanigan MD, Crompton LA, Reynolds CK, Wray-Cahen D, Lomax MA, France J. An integrative model of amino acid metabolism in the liver of the lactating dairy cow. J Theor Biol. (2004) 228:271–89. doi: 10.1016/j.jtbi.2004.01.010
7. Fleming AJ, Lapierre H, Martineau R, White RR, Hanigan MD. Modeling portal-drained viscera and liver fluxes of essential amino acids in dairy cows. J Dairy Sci. (2019) 102:10964–82. doi: 10.3168/jds.2019-16302
8. Berthiaume R, Dubreuil P, Stevenson M, McBride BW, Lapierre H. Intestinal disappearance and mesenteric and portal appearance of amino acids in dairy cows fed ruminally protected methionine. J Dairy Sci. (2001) 84:194–203. doi: 10.3168/jds.S0022-0302(01)74469-4
9. Martineau R, Ouellet DR, Kebreab E, White RR, Lapierre H. Relationships between postruminal casein infusion and milk production, and concentrations of plasma amino acids and blood urea in dairy cows: a multilevel mixed-effects meta-analysis. J Dairy Sci. (2017) 100:8053–71. doi: 10.3168/jds.2016-11813
10. Omphalius C, Lapierre H, Guinard-Flament J, Lamberton P, Bahloul L, Lemosquet S. Amino acid efficiencies of utilization vary by different mechanisms in response to energy and protein supplies in dairy cows: study at mammary-gland and whole-body levels. J Dairy Sci. (2019) 102:9883–901. doi: 10.3168/jds.2019-16433
11. Clark JH. Lactational Responses to Postruminal administration of proteins and amino acids. J Dairy Sci. (1975) 58:1178–97. doi: 10.3168/jds.S0022-0302(75)84696-0
12. Mepham TB. Amino acid utilization by lactating mammary gland. J Dairy Sci. (1982) 65:287–98. doi: 10.3168/jds.S0022-0302(82)82191-7
13. King KJ, Bergen WG, Sniffen CJ, Grant AL, Grieve DB, King VL, et al. An assessment of absorbable lysine requirements in lactating cows. J Dairy Sci. (1991) 74:2530–9. doi: 10.3168/jds.S0022-0302(91)78430-0
14. Schwab CG, Bozak CK, Whitehouse NL, Olson VM. Amino acid limitation and flow to the duodenum at four stages of lactation. 2 extent of lysine limitation. J Dairy Sci. (1992) 75:3503–18. doi: 10.3168/jds.S0022-0302(92)78126-0
15. Robinson PH, Swanepoel N, Shinzato I, Juchem SO. Productive responses of lactating dairy cattle to supplementing high levels of ruminally protected lysine using a rumen protection technology. Anim Feed Sci Technol. (2011) 168:30–41. doi: 10.1016/j.anifeedsci.2011.03.019
16. Lee C, Hristov AN, Cassidy TW, Heyler KS, Lapierre H, Varga GA, et al. Rumen-protected lysine, methionine, and histidine increase milk protein yield in dairy cows fed a metabolizable protein-deficient diet. J Dairy Sci. (2012) 95:6042–56. doi: 10.3168/jds.2012-5581
17. Lapierre H, Milne E, Renaud J, Lobley GE. Lysine utilization by the mammary gland. In: Souffrant WB, Metges CC, editors. Progress in Research on Energy and Protein Metabolism. Rostock: EAAP Publication No. 109 (2003), p. 777–80.
18. Weekes TL, Luimes PH, Cant JP. Responses to amino acid imbalances and deficiencies in lactating dairy cows. J Dairy Sci. (2006) 89:2177–87. doi: 10.3168/jds.S0022-0302(06)72288-3
19. Jeyapalan AS, Orellana RA, Suryawan A, O'Connor PM, Nguyen HV, Escobar J, et al. Glucose stimulates protein synthesis in skeletal muscle of neonatal pigs through an Ampk- and Mtor-independent process. Am J Physiol Endocrinol Metab. (2007) 293:E595–603. doi: 10.1152/ajpendo.00121.2007
20. Lynch CJ, Patson BJ, Anthony J, Vaval A, Jefferson LS, Vary TC. Leucine is a direct-acting nutrient signal that regulates protein synthesis in adipose tissue. Am J Physiol Endocrinol Metab. (2002) 283:E503–13. doi: 10.1152/ajpendo.00084.2002
21. Williamson DH. The lactating mammary gland of the rat and the starved-refed transition: a model system for the study of the temporal regulation of substrate utilization. Biochem Soc Trans. (1990) 18:853–6. doi: 10.1042/bst0180853
22. Chaiyabutr N, Faulkner A, Peaker M. Effects of exogenous glucose on glucose metabolism in the lactating goat in vivo. Br J Nutr. (1983) 49:159–65. doi: 10.1079/BJN19830021
23. Toerien CA, Trout DR, Cant JP. Nutritional stimulation of milk protein yield of cows is associated with changes in phosphorylation of mammary eukaryotic initiation factor 2 and ribosomal S6 kinase 1. J Nutr. (2010) 140:285–92. doi: 10.3945/jn.109.114033
24. Liu W, Xia F, Hanigan MD, Lin XY, Yan ZG, White RR, et al. Short-term lactation and mammary metabolism responses in lactating goats to graded removal of methionine from an intravenously infused complete amino acid mixture. J Dairy Sci. (2019) 102:4094–104. doi: 10.3168/jds.2018-15643
25. Mineo H, Oyamada T, Akiyama M, Yasuda T, Kato S. A new method of catheterization of the hepatic and portal veins in sheep for long-term blood sampling. Small Rumin Res. (1991) 5:293–300. doi: 10.1016/0921-4488(91)90133-B
27. Bequette BJ, Backwell FR, MacRae JC, Lobley GE, Crompton LA, Metcalf JA, et al. Effect of intravenous amino acid infusion on leucine oxidation across the mammary gland of the lactating goat. J Dairy Sci. (1996) 79:2217–24. doi: 10.3168/jds.S0022-0302(96)76598-0
28. Annison EF, Linzell JL. The oxidation and utilization of glucose and acetate by the mammary gland of the goat in relation to their over-all metabolism and to milk formation. J Physiol. (1964) 175:372–85. doi: 10.1113/jphysiol.1964.sp007522
29. Katz ML, Bergman EN. Simultaneous measurements of hepatic and portal venous blood flow in the sheep and dog. Am J Physiol. (1969) 216:946–52. doi: 10.1152/ajplegacy.1969.216.4.946
30. Cant JP, DePeters EJ, Baldwin RL. Mammary amino acid utilization in dairy cows fed fat and its relationship to milk protein depression. J Dairy Sci. (1993) 76:762–74. doi: 10.3168/jds.S0022-0302(93)77400-7
31. Huntington GB, Zetina EJ, Whitt JM, Potts W. Effects of dietary concentrate level on nutrient absorption, liver metabolism, and urea kinetics of beef steers fed isonitrogenous and isoenergetic diets. J Anim Sci. (1996) 74:908–16. doi: 10.2527/1996.744908x
32. Hanigan MD, France J, Wray-Cahen D, Beever DE, Lobley GE, Reutzel L, et al. Alternative models for analyses of liver and mammary transorgan metabolite extraction data. Br J Nutr. (1998) 79:63–78. doi: 10.1079/BJN19980010
33. Misciatteilli L, Kristensen VF, Vestergaard M, Welsbjerg MR, Sejrsen K, Hvelplund T. Milk production, nutrient utilization, and endocrine responses to increased postruminal lysine and methionine supply in dairy cows. J Dairy Sci. (2003) 86:275–86. doi: 10.3168/jds.S0022-0302(03)73606-6
34. Lapierre H, Doepel L, Milne E, Lobley GE. Responses in mammary and splanchnic metabolism to altered lysine supply in dairy cows. Animal. (2009) 3:360–71. doi: 10.1017/S1751731108003571
35. Wang C, Liu HY, Wang YM, Yang ZQ, Liu JX, Wu YM, et al. Effects of Dietary Supplementation of methionine and lysine on milk production and nitrogen utilization in dairy cows. J Dairy Sci. (2010) 93:3661–70. doi: 10.3168/jds.2009-2750
36. Paz HA, de Veth MJ, Ordway RS, Kononoff PJ. Evaluation of rumen-protected lysine supplementation to lactating dairy cows consuming increasing amounts of distillers dried grains with solubles. J Dairy Sci. (2013) 96:7210–22. doi: 10.3168/jds.2013-6906
37. Paz HA, Kononoff PJ. Lactation responses and amino acid utilization of dairy cows fed low-fat distillers dried grains with solubles with or without rumen-protected lysine supplementation. J Dairy Sci. (2014) 97:6519–30. doi: 10.3168/jds.2014-8315
38. Lee C, Giallongo F, Hristov AN, Lapierre H, Cassidy TW, Heyler KS, et al. Effect of dietary protein level and rumen-protected amino acid supplementation on amino acid utilization for milk protein in lactating dairy cows. J Dairy Sci. (2015) 98:1885–902. doi: 10.3168/jds.2014-8496
39. Fraser DL, Rskov ER, Whitelaw FG, Franklin MF. Limiting amino acids in dairy cows given casein as the sole source of protein. Livest Prod Sci. (1991) 28:235–52. doi: 10.1016/0301-6226(91)90145-G
40. Hippen AR, She P, Young JW, Beitz DC, Lindberg GL, Richardson LF, et al. Metabolic responses of dairy cows and heifers to various intravenous dosages of glucagon. J Dairy Sci. (1999) 82:1128–38. doi: 10.3168/jds.S0022-0302(99)75336-1
41. She P, Hippen AR, Young JW, Lindberg GL, Tucker RW. Metabolic responses of lactating dairy cows to 14-day intravenous infusions of glucagon. J Dairy Sci. (1999) 82:1118–27. doi: 10.3168/jds.S0022-0302(99)75335-X
42. Lacasse P, Lollivier V, Dessauge F, Bruckmaier RM, Ollier S, Boutinaud M. New developments on the galactopoietic role of prolactin in dairy ruminants. Domest Anim Endocrinol. (2012) 43:154–60. doi: 10.1016/j.domaniend.2011.12.007
43. Forsyth IA, Lee PD. Bromocriptine treatment of periparturient goats: long-term suppression of prolactin and lack of effect on lactation. J Dairy Res. (1993) 60:307–17. doi: 10.1017/S0022029900027655
44. Akers RM, Bauman DE, Capuco AV, Goodman GT, Tucker HA. Prolactin regulation of milk secretion and biochemical differentiation of mammary epithelial cells in periparturient cows. Endocrinology. (1981) 109:23–30. doi: 10.1210/endo-109-1-23
45. Akers RM, Bauman DE, Goodman GT, Capuco AV, Tucker HA. Prolactin regulation of cytological differentiation of mammary epithelial cells in periparturient cows. Endocrinology. (1981) 109:31–40. doi: 10.1210/endo-109-1-31
46. Knight CH, Foran D, Wilde CJ. Interaction between autocrine and endocrine control of milk yield: thrice-daily milking and bromocriptine-treated goats. J Reprod Fertil. (1990) 5:30–36.
47. Varvikko T, Vanhatalo A, Jalava T, Huhtanen P. Lactation and metabolic responses to graded abomasal doses of methionine and lysine in cows fed grass silage diets. J Dairy Sci. (1999) 82:2659–73. doi: 10.3168/jds.S0022-0302(99)75523-2
48. Hanigan MD, France J, Crompton LA, Bequette BJ. Evaluation of a representation of the limiting amino acid theory for milk protein synthesis. In: McNamara JP, France J, Beever DE, editors. Modeling Nutrient Utilization in Farm Animals. Oxfordshire: CABI Publishing (2000), p. 127–144. doi: 10.1079/9780851994499.0127
49. De Visser H, Valk H, Klop A, Van der Meulen J, Bakker JG, Huntington GB. Nutrient fluxes in splanchnic tissue of dairy cows: influence of grass quality. J Dairy Sci. (1997) 80:1666–73. doi: 10.3168/jds.S0022-0302(97)76098-3
50. Bach A, Huntington GB, Calsamiglia S, Stern MD. Nitrogen metabolism of early lactation cows fed diets with two different levels of protein and different amino acid profiles. J Dairy Sci. (2000) 83:2585–95. doi: 10.3168/jds.S0022-0302(00)75152-6
51. Raggio G, Pacheco D, Berthiaume R, Lobley GE, Pellerin D, Allard G, et al. Effect of level of metabolizable protein on splanchnic flux of amino acids in lactating dairy cows. J Dairy Sci. (2004) 87:3461–72. doi: 10.3168/jds.S0022-0302(04)73481-5
52. Huntington GB. Energy metabolism in the digestive tract and liver of cattle: influence of physiological state and nutrition. Reprod Nutr Dev. (1990) 30:35–47. doi: 10.1051/rnd:19900103
53. Bequette BJ, Hanigan MD, Calder AG, Reynolds CK, Lobley GE, MacRae JC. Amino acid exchange by the mammary gland of lactating goats when histidine limits milk production. J Dairy Sci. (2000) 83:765–75. doi: 10.3168/jds.S0022-0302(00)74939-3
54. Cant JP, Berthiaume R, Lapierre H, Luimes PH, Mcbride BW, Pacheco D. Responses of the bovine mammary glands to absorptive supply of single amino acids. Can J Anim Sci. (2003) 83:341–55. doi: 10.4141/A02-077
55. Laughlin MH, Korzick DH. Vascular smooth muscle: integrator of vasoactive signals during exercise hyperemia. Med Sci Sports Exerc. (2001) 33:81–91. doi: 10.1097/00005768-200101000-00014
56. MacRae JC, Bruce LA, Brown DS, Calder AG. Amino acid use by the gastrointestinal tract of sheep given lucerne forage. Am J Physiol. (1997) 273:G1200–7. doi: 10.1152/ajpgi.1997.273.6.G1200
57. Rulquin H, Raggio G, Lapierre H, Lemosquet S. Relationship between intestinal supply of essential amino acids and their mammary metabolism in the lactating dairy cow. In: Ortigues-Marty I,editor. Energy & Protein Metabolism & Nutrition. Vichy: EAAP publication No. 124. (2007), p. 587–8.
58. Raggio G, Lemosquet S, Lobley GE, Rulquin H, Lapierre H. Effect of casein and propionate supply on mammary protein metabolism in lactating dairy cows. J Dairy Sci. (2006) 89:4340–51. doi: 10.3168/jds.S0022-0302(06)72481-X
Keywords: lysine, lactating goats, amino acids, splanchnic tissues, mammary glands
Citation: Li Y, Lin X, Liu C, Hu Z, Hou Q and Wang Z (2022) Assessing Amino Acid Metabolism in Splanchnic Tissues and Mammary Glands to Short-Term Graded Removal of Lys From an Abomasal-Infused Amino Acid Mixture in Lactating Goats. Front. Vet. Sci. 9:929587. doi: 10.3389/fvets.2022.929587
Received: 27 April 2022; Accepted: 17 May 2022;
Published: 17 June 2022.
Edited by:
Bing Dong, China Agricultural University, ChinaCopyright © 2022 Li, Lin, Liu, Hu, Hou and Wang. This is an open-access article distributed under the terms of the Creative Commons Attribution License (CC BY). The use, distribution or reproduction in other forums is permitted, provided the original author(s) and the copyright owner(s) are credited and that the original publication in this journal is cited, in accordance with accepted academic practice. No use, distribution or reproduction is permitted which does not comply with these terms.
*Correspondence: Zhonghua Wang, zhwang@sdau.edu.cn