Impact of soil types and root exudates on cadmium and petroleum hydrocarbon phytoremediation by Sorghum sudanense, Festuca arundinace, and Lolium perenne
- College of Chemistry and Environmental Engineering, Yangtze University, Jingzhou, China
Objective: With the development of industrialization, soils around the world have been polluted by heavy metals and oil to different degrees in recent years, and soil remediation has become a global problem. Phytoremediation has a wide application prospect because of its environmental friendliness and easy availability of materials.
To explore the effects of soil types and root exudates on the removal of cadmium and petroleum hydrocarbon in soils.
Method: A pot experiments with three soil types (sandy, loamy and clayey) of the Changning-Weiyuan area of Sichuan province and three root exudates (citric acid, glycine, and maltose) were carried out using Sorghum sudanense (Piper) Stapf., Lolium perenne L., and Festuca arundinacea L. as test materials. Plants were grown in soils contaminated by cadmium and petroleum at different concentrations.
Result: The biomass of S. sudanense, the translocation ratio and removal rate of cadmium in S. sudanense decreased gradually with increasing soil cadmium concentration. The promotion effects of the three root exudates on S. sudanense were in the following order: citric acid > glycine > maltose. At the same cadmium pollution conditions, the biomass levels of S. sudanense in sandy, loamy, and clayey soils were in the following order: clayey soil > loamy soil > sandy soil. On the contrary, the concentration, translocation ratio, and removal rate of cadmium in S. sudanense grown in the different soils treated with root exudates were in the following order: sandy soil > loamy soil > clayey soil. Under the three soil conditions, the fresh weight of F. arundinacea (0.36 ~ 0.68 g) and S. sudanense (0.51 ~ 0.99 g) increased significantly (p < 0.05). The total petroleum hydrocarbons degradation efficiencies of F. arundinacea, L. perenne, and S. sudanense were high in sandy soil (34.27% ~ 60.52%). Changing the type of root exudate had a significant impact on the degradation of total petroleum hydrocarbons in sandy soil (p < 0.05).
Conclusion: This study showed that soil types impacted the accumulation of cadmium and petroleum in plants. Phytoremediation of cadmium and petroleum contaminated soil could be enhanced by the application of root exudates. This study recommend that the method is suitable for field remediation of soils contaminated with mild cadmium and petroleum hydrocarbons.
Introduction
Soil is an important component of the biosphere and lithosphere interface environment. As a limited natural resource, soil is vital to flora, fauna, and human activities (Sylvain et al., 2016). Followed by an extensive period of time, biology, topography, and climate, parent material in the lithosphere develops into soils with various properties (Pan et al., 2020). Soil texture is among the vital factors concerning the quality and productivity of soil (Pan et al., 2020). As per the international classification standards for soil texture types, soil can be divided into sandy, loamy, and clayey (Li et al., 2020). The physical and chemical properties, along with parameters of different soil texture types vary greatly, resulting in their ability to form heavy metal complexes and unequal influences on plant growth and surrounding microorganisms (Marschner et al., 1996).
Soil pollution is among the most important threats to soil health (Gómez-Sagasti et al., 2016). Among the various pollutants, the risk of Cadmium (Cd) and petroleum hydrocarbon pollution has become the focus of our attention. Cd is recognized as one of the most toxic trace elements in soils (Mao et al., 2022). Due to high mobility and bioavailability of Cd, the cycle of it in the soil–plant system has a high global correlation. Reports have suggested that approximately 1.0 × 106 t of Cd is released annually into the environment across the world. In China, the over-standard rate of soil Cd is 7.0% compared with the soil environmental quality standard, while the greater over-standard rate is of most concern (Hu et al., 2020). As an important source of energy in modern society, petroleum occupies a very important position in the national economy. However, with the rapid development of oil drilling, the petrochemical industry, and the wide use of petroleum products, the soil pollution of petroleum has become a serious environmental problem (Liu et al., 2020a). Petroleum hydrocarbons could affect DNA, disrupt normal functions of cellular membranes threatening the life cycle of organisms (Parnian et al., 2022). Long-term petroleum pollution not only degrades the soil function but also endangers the health of human and ecosystem.
Research on soil remediation mainly focuses on pollutant removal efficiency, soil remediation cycle, and costs (Awa and Hadibarata, 2020). However, the soil environment and nutrients also affect successful soil remediation. Specific soil properties including clay content, pH, cation exchange capacity, total nitrogen, etc., are confirmed to affect heavy metal phytoavailability (Farrag et al., 2012). Cao et al. (2021) investigated the influence of soil properties on Cd concentrations in the oilseed rape and S. alfredii concluding that the magnitude of Cd accumulation differed in different soil types and was related to available phosphorus, pH, soil organic matter, available potassium, silt and sand. Wang et al. (2021a) studied the effects of soil types on the growth and cadmium and zinc absorption of Sedum plumbizincicola. The shoot biomass of plants in clayey soil was significantly higher than that in sandy soil, but the plant root activity was higher in sandy soil. Moreover, the cadmium ion and zinc concentrations in the root were higher. Wang et al. (2021b) discussed the yield and quality of wheat under three different soil conditions through a pot experiment and found that the grain and protein yields of wheat were in the following order: black soil > alluvial soil.
Phytoremediation is a set of techniques that use plants and related microorganisms to remove, detoxify, and retain organic and inorganic pollutants in order to rehabilitate contaminated sites (Madejón et al., 2018). As a low-cost, eco-friendly, and sustainable strategy method, phytoremediation can efficiently transfer heavy metals from soil to plant branches and leaves (Tiecher et al., 2016; Khan et al., 2021). Plant roots can permeate the soil through means of vegetation cover allowing for soil remediation (Zhao et al., 2019). A large number of complex interactions between roots and soils can change the properties and enhance the biological composition of the soil (Hodge et al., 2009). The process of phytoremediation involves different biochemical and physiological mechanisms facilitated by absorption, accumulation, sequestration, transport, and degradation. Furthermore, plants can metabolize hazardous organic contaminants into nontoxic or comparatively fewer in toxic forms (Tripathi et al., 2020a). The key to phytoremediation lies in the selection of hyperaccumulators. Sorghum sudanense (Piper) Stapf. (S. sudanense) with a vigorous root system and strong tolerance of both PAHs and heavy metals demonstrating a potential for phytoremediation (Liu et al., 2020b). Festuca arundinacea L with rapid growth, high rhizosphere microbial density and rhizosphere activity is an ideal choice for the phytoremediation of petroleum hydrocarbon-contaminated soils (Hou et al., 2021). Lolium perenne L. is a great resistant species to diesel pollutants (Wyszkowska et al., 2019). Selecting cultivar with high remediation efficiency is a promising way to promote phytoremediation (Hou et al., 2021). Besides, root exudates include various carbon sources like primary metabolites such as phenolic acids, organic acids, sugars, amino acids and secondary metabolites compounds that influence the bioavailability and biodegradation of pollutants (Al-Ali et al., 2018; Liao et al., 2021). Furthermore, the diversity of root exudates results in the changes of soil microbial composition, soil organic matter and the physical structure of soil. These changes results unto modulations in various associated soil physiological processes (Tripathi et al., 2020b). Previous studies mainly targeted on organic acids, and the other categories of root secretion mechanisms and influencing factors are less reported. Amino acids and sugars can be widely detected in plant tissue and root secretion, but the effect of these compounds on pollutants did not attract researchers’ attention.
In our previous experiment, we choose five different kinds of root exudates including citric acid (organic acid), glycine (amino acid), vanillic acid (phenol), stearic acid (fatty acid), and maltose (saccharide) with plants to compare their efficiency on remediation of cadmium and petroleum contaminated soils. The result showed that citric acid, glycine, and maltose had the best removal effect on cadmium, while glycine and maltose had the best removal effect on petroleum among the five root exudates. In this experiment, three soil types (sandy, loamy, and clayey) and three kinds of root exudates (organic acids, amino acids, and saccharides represented by citric acid, glycine, and maltose respectively) were used as research objects to explore the effect of root exudates on the removal of cadmium and petroleum hydrocarbon in soil under different soil conditions. Two hypotheses were developed: (1) Root exudates could hasten plant growth and facilitate the removal of cadmium and petroleum hydrocarbons from contaminated soil; (2) Different soil types could vary with the plant growth and the effect of phytoremediation on contaminated soil. Therefore, the biomass, height, and fresh weight of plants grown in the contaminated soil were measured. Cadmium concentration in plants was determined, and the translocation ratio and removal rates of cadmium were also calculated. The degradation rate of petroleum hydrocarbons was calculated by measuring the total amount of petroleum hydrocarbons in soil. The results will provide theoretical basis and technical support for phytoremediation in different polluted soils.
Experimental materials and methods
Materials
Climate condition
Three kinds of soil (sandy, clayey, and loamy; 0–20 cm) were selected from the pollution-free soil in the Changning-Weiyuan area of Sichuan province (104°34′34.5″E, 29°29′54.3″N). The climate of this area is subtropical monsoon warm and wet climate zone, perennial warm, wet and foggy. The average annual temperature is about 18°C, and the average annual rainfall is 1141.7 mm.
Soil sampling
Soil samples were carried out using a 5 cm diameter auger. Ten sub-samples per experimental location were collected randomly to form one composite sample, air-dried and sieved (0.5 mm). Soil samples were analyzed for soil texture, pH, organic matter, total nitrogen, available phosphorous, exchangeable potassium content, bulk density, and cadmium content, using standard methods (Estefan et al., 2013). Soil pH was determined in soil–water (1:2) suspension using a pH meter (Equipment model: PHS-3E; Equipment serial number: SEP-SH-J792). Soil texture was determined by the Hydrometer method. Soil bulk density was measured by the core cylinder method (Electronic balance, ME2002E/02, SEP-H-J273). The wet oxidation method followed by titration was used to measure organic carbon (Teflon core burette, 25 ml, SEP-SH-J606). Total nitrogen (TN) was determined after wet digestion by the Kjeldahl method, and available phosphorus (AP) using an Ultraviolet–visible spectrophotometer at 882-nm wavelength (Cary100; SEP-SH-J123). Exchangeable potassium (EK) was measured by using a Flame atomic absorption spectrophotometer (AA280FS, SEP-SH-J703). Cadmium was determined by Atomic Absorption Spectrophotometer (WYS2400). The amount of total petroleum hydrocarbons in soil was quantified gravimetrically (Zhang et al., 2019). The basic physical and chemical characteristics of experimental soils are shown in Table 1.
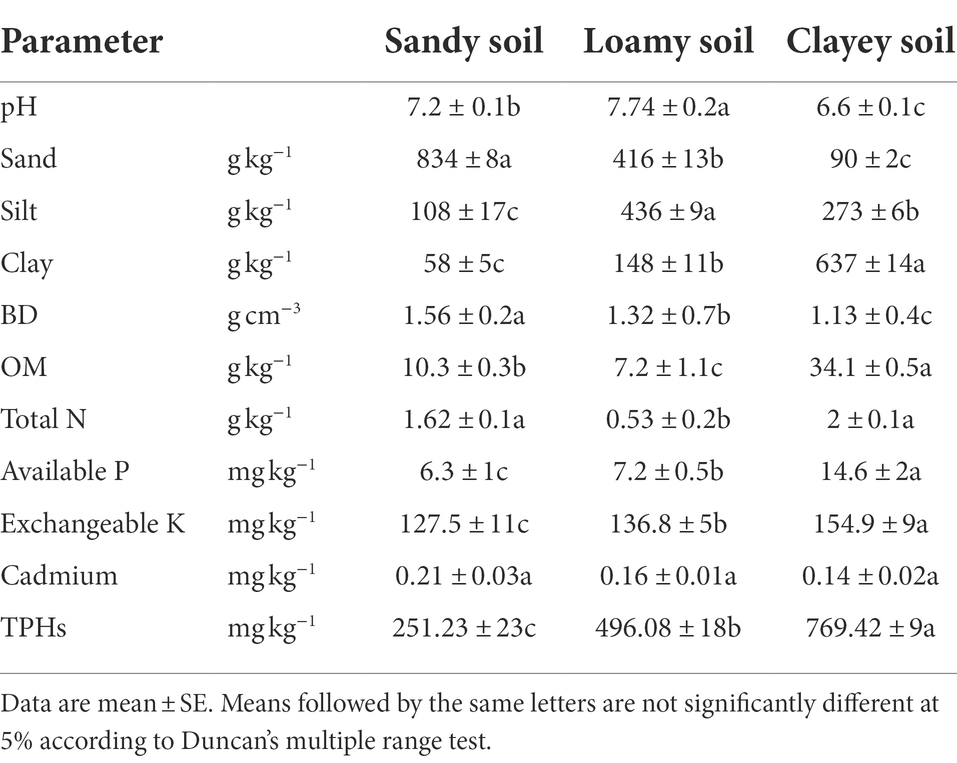
Table 1. Physico-chemical characteristics of experimental soils (0–20 cm), including pH, Sand, Silt, Clay, Bulk Density (BD), Organic Matter (OM), Total Nitrogen (Total N), Available Phosphorus (Available P), Exchangeable Potassium (Exchangeable K), Cadmium and Total Petroleum Hydrocarbons (TPHs).
Reagents
The following reagents were purchased from Sinopharm Chemical Reagent Co., Ltd. (Tianjin): citric acid (AR), glycine (AR), maltose (AR), 65% nitric acid (AR), hydrofluoric acid (AR), and perchloric acid (AR). S. sudanense, F. arundinacea, and L. perenne seeds were purchased from Huazhong Agricultural University. Deionized water was used in the experiment.
Test design
Removal of cadmium from the soil by root exudates under different soil conditions
Two pieces of filter paper were placed in each Petri dish with a diameter of 90 mm. Using the improved Hoagland solution as a culture medium, 20 S. sudanense seeds were placed in each Petri dish and cultured in a climate chamber. The nutrient element composition of the improved Hoagland solution (Chen et al., 2020) was as follows: 5.0 mmol/l Ca2+, 5.0 mmol/l K+, 1.0 mmol/l KH2PO4, 1.0 mmol/l Mg2+, 1.0 mmol/l Zn2+, and 1.0 mmol/l Cu2+. The temperature was maintained at 25 ± 0.5°C, the humidity was at 75 ± 5%, light intensity was at 80 ± 1 lx, and day: night photoperiod was 16:8 h. Seedlings were transplanted when they had 2–3 true leaves (Aram et al., 2021).
500 g of each prepared sandy, loamy, and clayey soil was put into flowerpots. The following is according to a study on cadmium concentrations in polluted soil that used phytoremediation for cleaning (Nouri and Hashempour, 2021). CdCl2·5/2H2O (0.2036 g) was dissolved in a beaker to achieve a fixed volume in a 1 L volumetric flask. Serial dilutions of stock solution were performed to obtain 0.1 mg mL−1 of cadmium ion aqueous solution. Cadmium ion solutions at 5, 25, and 50 ml were mixed evenly with these soils to prepare 1, 5, and 10 mg kg−1 cadmium ion-contaminated soils, respectively (Arshad et al., 2017). All the prepared soils were air-dried for 1 week to maintain soil equilibrium before the use in the pot experiment.
Three experimental groups (Groups C: citric acid; G: glycine; M: maltose) and a control group (N: natural degradation) were set up for sandy, clayey, and loamy soil with cadmium ion concentrations of 10 mg kg−1, respectively. Ten seedlings were transplanted into each pot by hand at 2–3 cm depth. For C, G and M treatments, 20 ml citric acid, glycine, and maltose solutions at 50 mmol L−1 were added into corresponding pots, respectively. The corresponding solution (20 ml) and water (60 ml) were replenished daily. The control group in this experiment were 10 mg kg−1 cadmium ion-contaminated soils without planting grass or adding root exudates. There were three replicates for each test. After 40 days of treatment, the S. sudanense plants were harvested, washed repeatedly with deionized water, and dried in an oven at 104°C for 4 h until a constant weight was achieved. The dried plant samples were cut into roots and leaves-stems separately, and the biomass of these parts was weighed and calculated. The soil samples were air-dried and sieved (0.5 mm).
Removal of total petroleum hydrocarbons from the soil with root exudates under different soil conditions
The field investigation of the soil environment in the Changning-Weiyuan shale gas well site showed that the crude oil pollutant content was unevenly distributed, and the average value was about 12 g kg−1. Thus, the three kinds of dried clean soil (sandy, loamy, and clayey) were sieved (2 mm), and a certain mass of petroleum was weighed and dissolved in petroleum ether (each 1 kg of clean soil was thoroughly mixed with 500 ml of petroleum ether) to prepare a 12,000 mg kg−1 petroleum hydrocarbon-contaminated soil. The mixture was ventilated in a fume hood for 3 weeks to maintain soil equilibrium (Hussain et al., 2018).
Eighty-one plastic flowerpots with a height of 15 cm and a diameter of 12 cm were used, and each pot was filled with 600 g of soil. Glycine and maltose solutions at 2000 mg L−1 were added into corresponding pots, respectively. The seeds of F. arundinacea, L. perenne, and S. sudanense were germinated in the above ways. Ten morphologically uniform seedlings were transplanted into each flowerpot and placed randomly under natural light. Water was poured regularly into these pots to keep the soil moisture at approximately 65% and the temperature at 21°C–30°C. The soil samples of controls were watered only for natural degradation (no plants and root exudates were added under the control environment). There were nine treatment tests of each plant in the pot experiment (sandy soil, sandy soil + glycine 2,000 mg L−1, sandy soil + maltose 2,000 mg L−1; loamy soil, loamy soil + glycine 2,000 mg L−1, loamy soil + maltose 2,000 mg L−1; clayey soil, clayey soil + glycine 2,000 mg L−1, clayey soil + maltose 2,000 mg L−1), with three replicates for each test. The experiment was carried out in the greenhouse of the School of Chemical Engineering, Yangtze University. The trial lasted from 6 June 2021 to 12 August 2021, for a total of 67 days.
Measurement of plant indicators
The shoot and root parts of F. arundinacea, L. perenne, and S. sudanense were harvested on the 67th day of the growing period, and the plant height and fresh weight per plant were measured.
After germination, the seedling growth of different plants were observed under petroleum hydrocarbon pollution conditions in the different soil types. The growth status of plants was compared.
Measurement of cadmium concentration in roots and leaves-stems
First, the roots and leaves-stems of the plants were weighed and placed into a conical flask. Then, 20 ml of concentrated nitric acid was added. The components were thoroughly mixed and dissolved (temperature = 180°C). Hydrofluoric acid (10 ml) was added after the solution was volatilized and nearly dried. Then, 10 ml of perchloric acid was added when the solution evaporated and was nearly dried under constant stirring using a glass rod to prevent the splashing of the liquid. Finally, the inner wall of the conical flask was cleaned with 5% dilute nitric acid (Li et al., 2021; Rosa et al., 2021). The solution was filtered and placed into a 100 ml volumetric flask at a constant volume and eventually placed into the refrigerator at 4°C for testing. Cadmium concentration in roots and leaves-stems of plants was measured by Atomic Absorption Spectrophotometer (WYS2400).
Calculation of cadmium translocation ratio
The translocation ratio reflects the ability of plants to repair a certain pollutant, which is expressed as the ratio of pollutant concentrations in leaves-stems to those in roots. The calculation formula of cadmium translocation ratio (TF) is as follows:
Where, C1 is the content of cadmium in leaves-stems, and C2 is the content of cadmium in roots.
Calculation of cadmium removal rate
Soil samples (2 ± 0.01 g) were weighed in a conical flask, digested and measured as mentioned above. The calculation formula of cadmium removal rate (R) is as follows:
Where, C0 is the original content of cadmium in the soil, and C1 is the content of cadmium in the soil after restoration.
Measurement and calculation of total petroleum hydrocarbons In soil
Air-dried soil samples (5 g) were passed through a 2 mm screen and accurately weighed. They were then placed into a 50 ml centrifugal tube along with a 15 ml dichloromethane extractant, shaken and mixed evenly. Afterwards, ultrasonic extraction was performed for 20 min. Centrifugation was then performed at 3000 r min−1 for 10 min. The liquid and solid parts separated after letting the mixture stand, and the supernatant was poured into a triangle flask with a constant weight and baked. The above-mentioned steps were repeated thrice. The supernatant was placed into the triangle bottle, which was finally placed into a water bath at 60°C to steam out petroleum ether. Then, the supernatant was baked in the constant temperature box at 60°C for 1 h and eventually placed into the dryer to cool for 10 min and then weighed (Khan et al., 2017).
The calculation formula for the total petroleum hydrocarbons content (Mt) is expressed as follows:
Where, M1 is the mass of the triangle bottle, g; M2 is the total mass of petroleum hydrocarbon and triangle flask, g; m is the mass of soil samples, g; and P is the moisture content of soil sample, %.
Statistical analysis
The data was analyzed using IBM SPSS version 24.0 and Origin Pro version 8.0. The presented data are representing a means ± standard error of replication. The differences between treatments for each experiment variant were compared using one-way analysis of variance (ANOVA), followed by Duncan’s multiple range test at p ≤ 5% level. The correlation of root biomass, leaves-stems biomass, root Cd concentration, leaves-stems Cd concentration, and translocation ratio on different soil types of S. sudanense were analyzed by Person Correlation Analysis (p < 0.05). Soil types, root exudate types, and their interactions on fresh weight, plant height, and degradation rate of three plants were analyzed by the univariate multi-way ANOVA (p < 0.05).
Results and analysis
Effect of root exudates on the removal of cadmium in soil under different soil conditions
Effects of different soil types on the biomass of Sorghum sudanense
Table 1 shows the physico-chemical properties and contaminant contents in the soil samples. Analysis of pH showed that the sandy and loamy soil samples were mostly neutral in nature, while the clay soil was acidic. The contents of sand (F2,6 = 89424.571, p < 0.05), silt (F2,6 = 26896.333, p < 0.05) and clay (F2,6 = 17830.469, p < 0.05) were significantly different among the three soils. The bulk density of the soil samples was greater than 1, and the organic matter content of the clay soil was higher. High levels of essential plant nutrients, i.e., total nitrogen (TN) (0.53–2 g kg−1), Available phosphorous (AP) (6.3–14.6 g kg−1) and Exchangeable potassium (EK) (127.5–154.9 g kg−1) were found in the three soil samples. According to the risk screening value of Soil Environmental Quality Soil Pollution Risk Control Standard for Agricultural Land (GB 15618–2018): cadmium ≤0.30 mg kg−1. The cadmium content in the three soils is lower than the risk value. The content of petroleum hydrocarbon is also low, which can be regarded as clean soil.
Table 2 shows that the biomass of roots and leaves-stems of S. sudanense decreased with increasing cadmium ion concentration without the addition of root exudates. At a cadmium ion concentration of 1 mg kg−1, the biomass values of S. sudanense roots in sandy, loamy, and clayey soil were 0.35, 0.32, and 0.32 g, respectively. The biomass values of leaves-stems of S. sudanense in sandy, loamy, and clayey soils were 1.74, 1.77, and 1.89 g, respectively. The biomass of S. sudanense in sandy soil was similar to that in loamy soil, and the biomass of S. sudanense in clayey soil was higher than that in sandy and loamy soils (F2,6 = 40.500, p < 0.05). Clayey soil was more suitable for S. sudanense growth than the other soil types.
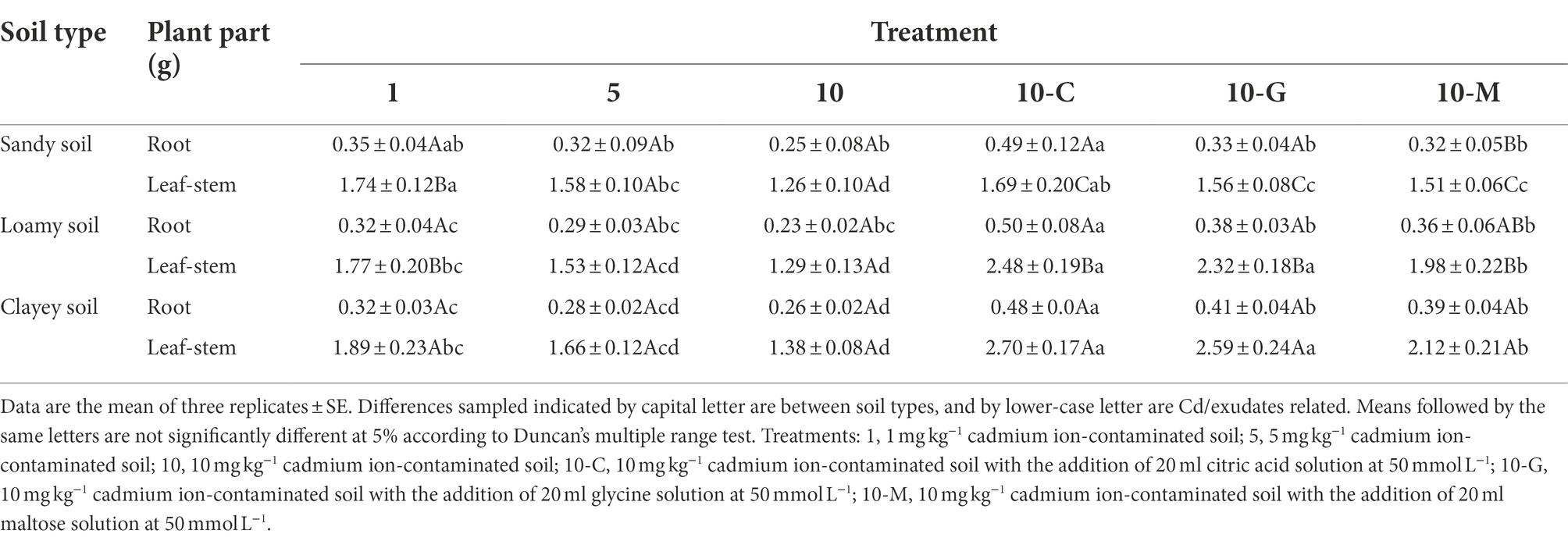
Table 2. Effects of root exudates on the biomass of root and leaf-stem of Sorghum sudanense under different soil types (g).
After adding 50 mmol L−1 of citric acid, glycine, and maltose to 10 mg kg−1 cadmium ion-contaminated soil (Table 2), the obtained biomass values of the root of S. sudanense in sandy soil were 0.49, 0.33, and 0.32 g, which were 96% (F5,12 = 12.339, p < 0.05), 32% (F5,12 = 12.339, p > 0.05), and 28% (F5,12 = 12.339, p > 0.05) higher than those obtained without the addition of root exudates (0.25 g), respectively. Citric acid promoted the growth of S. sudanense. Similar results were found in clayey and loamy soils. The addition of citric acid also promoted the biomass values of the S. sudanense leaves-stems. After adding 50 mmol L−1 of citric acid, glycine, and maltose to 10 mg kg−1 cadmium ion-contaminated clayey soil, the biomass values of S. sudanense leaves-stems were 2.70, 2.59, and 2.12 g, respectively. When the plant was grown in clayey soil, these values were 95.65% (F5,12 = 81.811, p < 0.05), 87.68% (F5,12 = 81.811, p < 0.05), and 53.62% (F5,12 = 81.811, p < 0.05) higher, respectively, compared with the plants grown without root exudate (1.38 g). The biomass values of the S. sudanense leaves-stems in clayey soil was significantly higher than that in sandy and loamy soil after each root exudate addition (citric acid: F2,6 = 390.600, p < 0.05; glycine: F2,6 = 56.794, p < 0.05; maltose: F2,6 = 124.176, p < 0.05).
In general, the promotion effect of the three root exudates at 50 mmol L−1 on S. sudanense was in the following order: citric acid > glycine > maltose. The growth of S. sudanense in three soil types with the addition of root exudates was in the following order: clayey soil > loamy soil > sandy soil.
Effects of different soil types on cadmium concentration in the roots of Sorghum sudanense
Figure 1 shows that the concentrations of cadmium in the roots of S. sudanense were 4.25, 10.56, and 15.80 mg kg−1 in sandy soil at 1, 5, and 10 mg kg−1 of cadmium ion, respectively. After adding 50 mmol L−1 of citric acid, glycine, and maltose (Figure 1), the concentrations of cadmium in the roots of S. sudanense in sandy soil polluted with 10 mg kg−1 of cadmium ion reached 24.82, 21.12, and 19.56 mg kg−1, respectively. Compared with the roots of plants grown without the addition of exudates, the concentrations of cadmium in the root of S. sudanense grown in sandy soil were higher by 57.09% (F5,12 = 78.364, p < 0.05), 33.67% (F5,12 = 78.364, p < 0.05), and 23.78% (F5,12 = 78.364, p < 0.05), respectively, possibly because of the activation effect of citric acid on cadmium ions in sandy soil was greater than those of glycine and maltose. The absorption of cadmium by the roots of S. sudanense in sandy soil was significantly higher than that in loamy and clayey soil after each root exudate addition (citric acid: F2,6 = 11841.524, p < 0.05; glycine: F2,6 = 1867.108, p < 0.05; maltose: F2,6 = 673.387, p < 0.05). The absorption of cadmium by the roots of S. sudanense in loamy soil was second only to that in sandy soil.
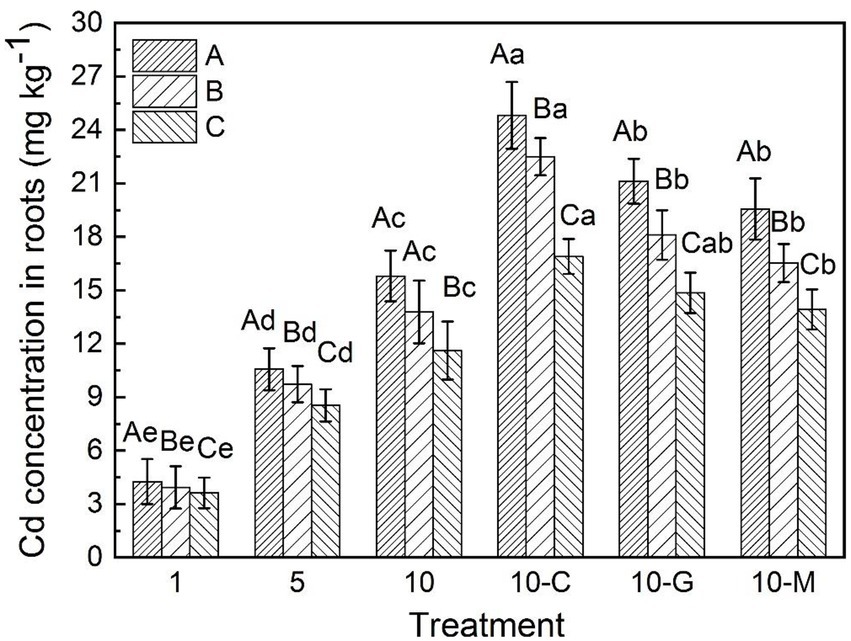
Figure 1. Cadmium concentration in roots of Sorghum sudanense under different soil types. The values shown are the mean ± SE (n = 3). Differences sampled indicated by capital letter are between soil types, and by lower-case letter are Cd/exudates related. The same letters among specific treatments are not significantly different according to Duncan’s (0.05). A, B, and C represent sandy, loamy, and clayey, respectivly. Treatments: 1, 1 mg kg−1 cadmium ion-contaminated soil; 5, 5 mg kg−1 cadmium ion-contaminated soil; 10, 10 mg kg−1 cadmium ion-contaminated soil; 10-C, 10 mg kg−1 cadmium ion-contaminated soil with the addition of 20 ml citric acid solution at 50 mmol L−1; 10-G, 10 mg kg−1 cadmium ion-contaminated soil with the addition of 20 ml glycine solution at 50 mmol L−1; 10-M, 10 mg kg−1 cadmium ion-contaminated soil with the addition of 20 ml maltose solution at 50 mmol L−1.
Effects of different soil types on cadmium concentration in leaves-stems of Sorghum sudanense
In sandy soil polluted with 1, 5, and 10 mg kg−1 of cadmium ion, the concentrations of cadmium in the leaves-stems of S. sudanense were 1.23, 3.64, and 6.55 mg kg−1, respectively (Figure 2). After adding 50 mmol L−1 of citric acid, glycine, and maltose, the concentrations of cadmium in the leaves-stems of S. sudanense in sandy soil polluted with 10 mg kg−1 of cadmium ion were 12.76, 9.07, and 7.97 mg kg−1, respectively (Figure 2). The concentrations of cadmium in the leaves-stems of S. sudanense were increased by 94.81% (F5,12 = 120.184, p < 0.05), 38.47% (F5,12 = 120.184, p < 0.05), and 21.68% (F5,12 = 120.184, p < 0.05), respectively. The addition of root exudates significantly promoted the cadmium concentration in the leaves and stems of S. sudanense, and citric acid had the best effect.
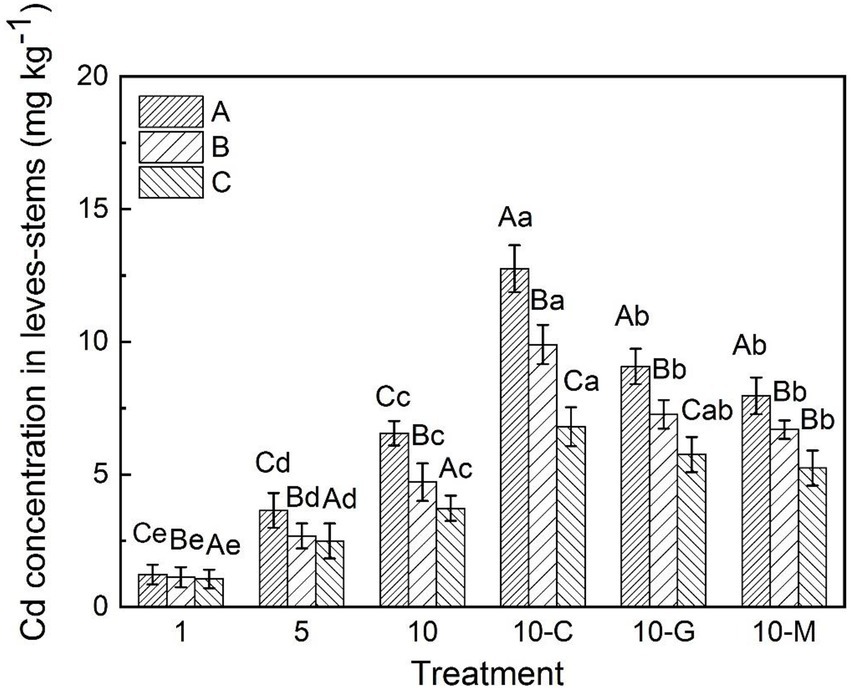
Figure 2. Cadmium concentration in leaves-stems of S. sudanense under different soil types. The values shown are the mean ± SE (n = 3). Differences sampled indicated by capital letter are between soil types, and by lower-case letter are Cd/exudates related. The same letters among specific treatments are not significantly different according to Duncan’s (0.05). A, B, and C represent sandy, loamy, and clayey, respectivly. Treatments: 1, 1 mg kg−1 cadmium ion-contaminated soil; 5, 5 mg kg−1 cadmium ion-contaminated soil; 10, 10 mg kg−1 cadmium ion-contaminated soil; 10-C, 10 mg kg−1 cadmium ion-contaminated soil with the addition of 20 ml citric acid solution at 50 mmol L−1; 10-G, 10 mg kg−1 cadmium ion-contaminated soil with the addition of 20 ml glycine solution at 50 mmol L−1; 10-M, 10 mg kg−1 cadmium ion-contaminated soil with the addition of 20 ml maltose solution at 50 mmol L−1.
The concentration of cadmium in the leaves-stems of S. sudanense in loamy and clayey soils also increased with the increasing cadmium concentration in soil. When the concentration of cadmium ion increased from 1 mg kg−1 to 10 mg kg−1 (Figure 2), the concentrations of cadmium in the leaves-stems of S. sudanense in loamy and clayey soils were 1.12–4.71 and 1.06–3.72 mg kg−1, respectively. In general, cadmium concentrations in the leaves-stems of S. sudanense in the three soil types were in the following order: sandy soil > loamy soil > clayey soil.
Translocation ratio of cadmium in Sorghum sudanense under different soil types
Figure 3 illustrates that the translocation ratios of cadmium in S. sudanense were 0.38, 0.34, and 0.33 at 1, 5, and 10 mg kg−1 cadmium ion concentrations in sandy soil, respectively. When the concentration of cadmium ion was at 10 mg kg−1 in sandy soil, the translocation ratios of cadmium in S. sudanense were 0.61, 0.50, and 0.45 after adding 50 mmol L−1 of citric acid, glycine, and maltose, respectively (Figure 3). These values were 84.85% (F5,12 = 22.556, p < 0.05), 51.52% (F5,12 = 22.556, p < 0.05), and 36.36% (F5,12 = 22.556, p < 0.05) higher than those obtained without the addition of root exudates, respectively. In conclusion, the addition of root exudates effectively promoted the transfer of cadmium from roots to leaves and stems.
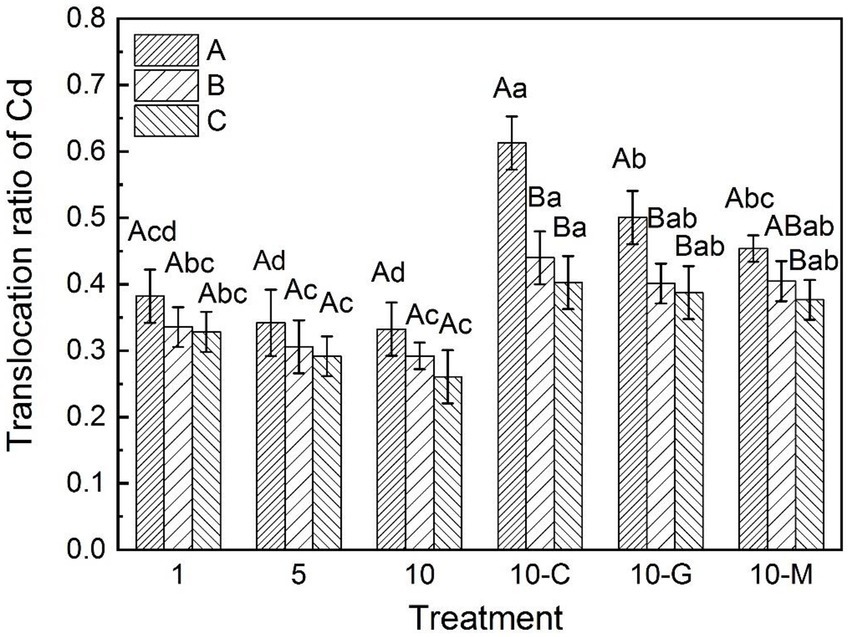
Figure 3. Translocation ratio of cadmium in S. sudanense in different soil types. The values shown are the mean ± SE (n = 3). Differences sampled indicated by capital letter are between soil types, and by lower-case letter are Cd/exudates related. The same letters among specific treatments are not significantly different according to Duncan’s (0.05). A, B, and C represent sandy, loamy, and clayey, respectivly. Treatments: 1, 1 mg kg−1 cadmium ion-contaminated soil; 5, 5 mg kg−1 cadmium ion-contaminated soil; 10, 10 mg kg−1 cadmium ion-contaminated soil; 10-C, 10 mg kg−1 cadmium ion-contaminated soil with the addition of 20 ml citric acid solution at 50 mmol L−1; 10-G, 10 mg kg−1 cadmium ion-contaminated soil with the addition of 20 ml glycine solution at 50 mmol L−1; 10-M, 10 mg kg−1 cadmium ion-contaminated soil with the addition of 20 ml maltose solution at 50 mmol L−1.
The translocation ratios of cadmium in S. sudanense in loamy and clayey soils decreased with increasing cadmium concentration in soil. When soil cadmium ion concentrations were 1, 5, and 10 mg kg−1, the translocation ratios of cadmium in S. sudanense in loamy soil were 0.34, 0.31, and 0.29, respectively (Figure 3). These values were 0.33, 0.29, and 0.26 in clayey soil, respectively. In general, cadmium translocation ratios in S. sudanense in the three soils were in the following order: sandy soil > loamy soil > clayey soil.
After adding citric acid, glycine, and maltose, the translocation ratios of cadmium in S. sudanense in loamy soil were 0.44, 0.40, and 0.40, respectively (Figure 3). These values were 0.40, 0.39, and 0.38 in clayey soil, respectively. Citric acid had the best promotion effect on cadmium transfer from roots to leaves and stems.
Cadmium removal rate in different soil types
Figure 4 shows that the removal rates of cadmium (1, 5, and 10 mg kg−1) in S. sudanense in sandy soil were 52.00, 44.10, and 34.65%, respectively. These values were 48.00, 37.10, and 29.65% in loamy soil and 42.00, 36.70, and 25.50% in clayey soil, respectively. In general, the cadmium removal rates of S. sudanense in the three soils under the same concentration of cadmium were in the following order: sandy soil > loamy soil > clayey soil.
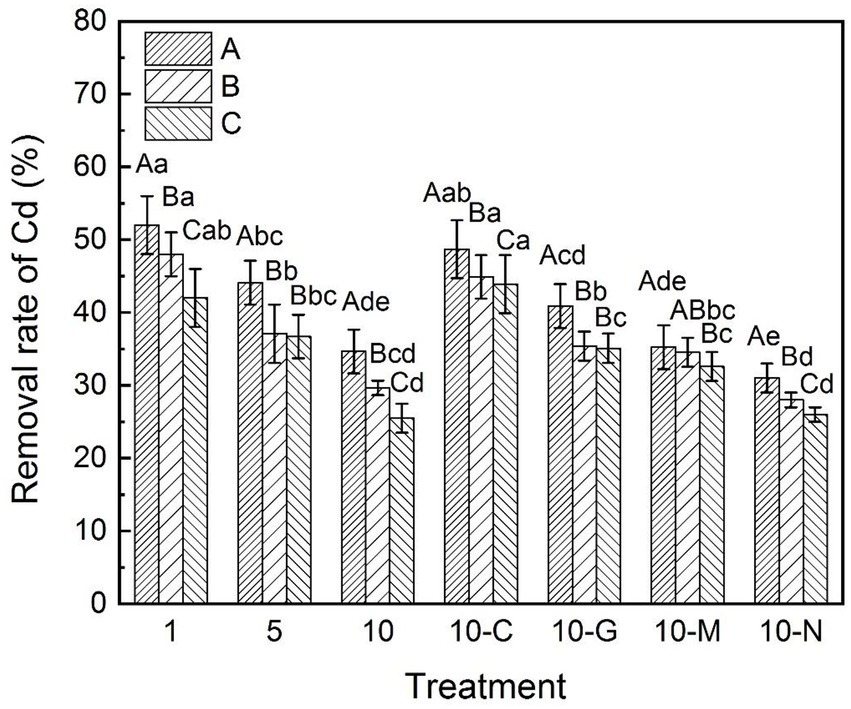
Figure 4. Removal rate of cadmium under different types of soil. The values shown are the mean ± SE (n = 3). Differences sampled indicated by capital letter are between soil types, and by lower-case letter are Cd/exudates related. The same letters among specific treatments are not significantly different according to Duncan’s (0.05). A, B, and C represent sandy, loamy, and clayey, respectivly. Treatments: 1, 1 mg kg−1 cadmium ion-contaminated soil; 5, 5 mg kg−1 cadmium ion-contaminated soil; 10, 10 mg kg−1 cadmium ion-contaminated soil; 10-C, 10 mg kg−1 cadmium ion-contaminated soil with the addition of 20 ml citric acid solution at 50 mmol L−1, 10-G, 10 mg kg−1 cadmium ion-contaminated soil with the addition of 20 ml glycine solution at 50 mmol L−1; 10-M, 10 mg kg−1 cadmium ion-contaminated soil with the addition of 20 ml maltose solution at 50 mmol L−1; 10-N, 10 mg kg−1 cadmium ion-contaminated soil without addition of root exudates.
After citric acid addition, the cadmium removal rate of S. sudanense significantly improved (Figure 4). In the three soil types, the cadmium ion removal rates of S. sudanense were 48.70% (sandy soil: F6,14 = 17.659, p < 0.05), 44.90% (loamy soil: F6,14 = 25.972, p < 0.05), and 43.90% (clayey soil: F6,14 = 19.876, p < 0.05), which were higher than those obtained when root exudates were not supplied. The cadmium removal rates of S. sudanense in loamy and clayey soils were lower, but the increase was most prominent. In sandy, loamy, and clayey soils, the cadmium removal rates of S. sudanense combined with glycine were 44.90, 35.40, and 34.52%, respectively (Figure 4). These values were 43.90, 35.10, and 32.60% with maltose, respectively. Citric acid was the best catalyst for removing cadmium from the soil, followed by glycine. The natural degradation effect of cadmium in the three soil conditions was significantly different (F2,6 = 150, p < 0.05) and lower than the removal effect of adding root exudates (sandy soil: F6,14 = 17.659, p < 0.05; loamy soil: F6,14 = 25.972, p < 0.05; clayey soil: F6,14 = 19.876, p < 0.05).
Correlation analysis of various indicators on different soil types of Sorghum sudanense
Correlation analysis shows (Table 3) that cadmium concentration in leaves-stems of S. sudanense is significantly correlated with the cadmium concentration in its root in three soil types (p < 0.01). In sandy soil, the translocation ratio of cadmium to S. sudanense was significantly correlated with root biomass (p < 0.05) and leaves-stems cadmium concentration (p < 0.05). The removal rate of cadmium was significantly correlated with the leaves-stems biomass (p < 0.05). In loamy soil, leaves-stems biomass and cadmium concentration in leaves-stems were significantly correlated with root biomass (p < 0.01) and cadmium concentration in the root (p < 0.01), respectively. The translocation ratio of cadmium to S. sudanense was significantly correlated with root biomass (p < 0.01), leaves-stems biomass (p < 0.01), and cadmium concentration in leaves-stems (p < 0.05). In clayey soil, the leaves-stems biomass of S. sudanense was significantly correlated with root biomass (p < 0.05), and the translocation ratio was significantly correlated with the root and leaves-stems biomass (p < 0.01).
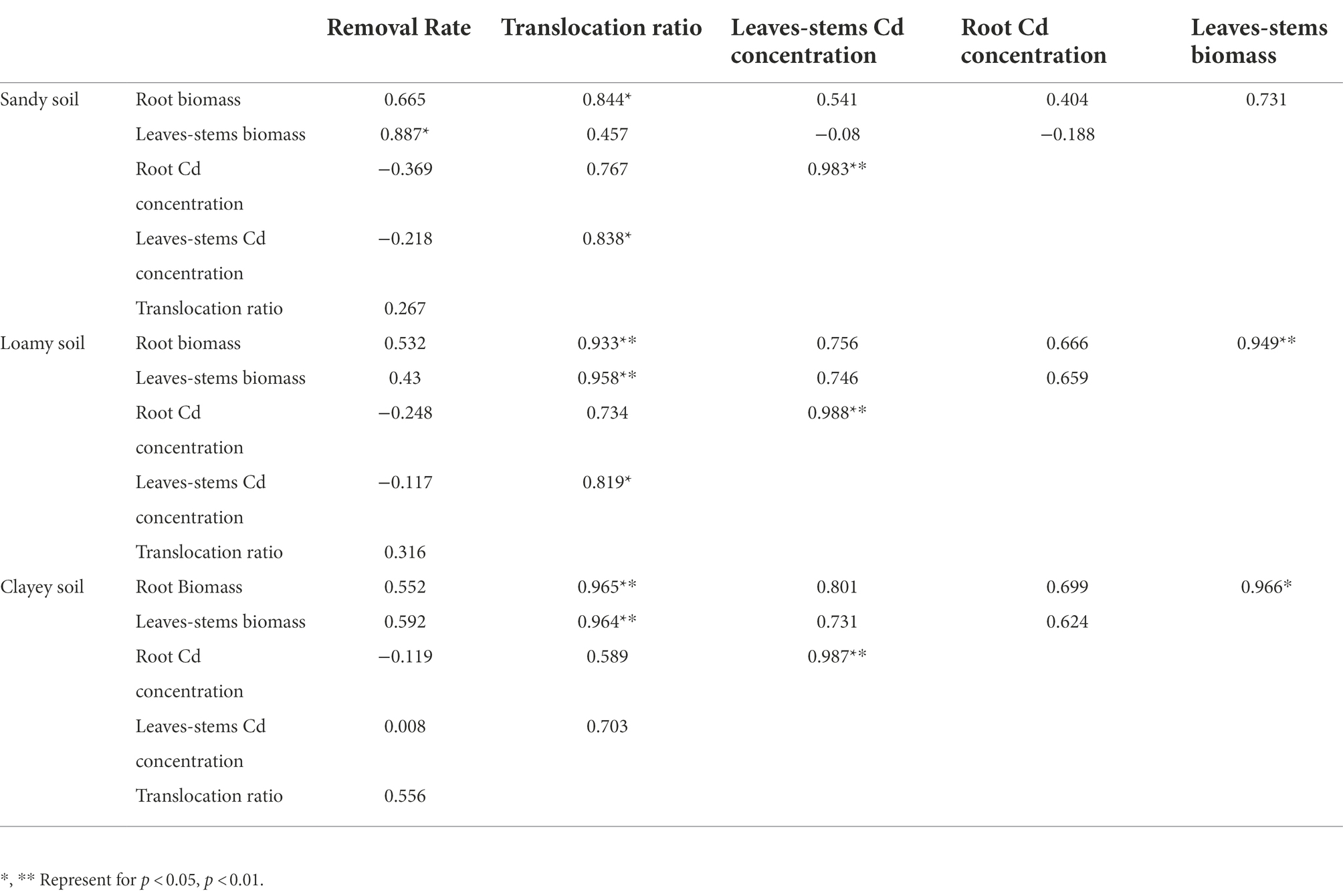
Table 3. Correlation analysis of various indicators on different soil types of Sorghum sudanense (Person correlation analysis).
Effects of root exudates on the removal of total petroleum hydrocarbons in soil under different soil conditions
According to Table 4, the fresh weight per plant of F. arundinacea and S. sudanense increased significantly in the three soil types. The fresh weight values per plant in the three soils were in the following order: sandy soil < clayey soil < loamy soil (F. arundinacea: F2,15 = 57.811, p < 0.05; S. sudanense: F2,15 = 57.902). Loamy soil was suitable for the growth of all three plants.
At 2000 mg L−1, glycine and maltose had little effect on the fresh weight of the three plants under the three soil conditions (Table 4). Thus, the average fresh weight values of plants treated with the two root exudates were obtained. The average fresh weight values of F. arundinacea were 0.375 and 0.525 g in sandy and clayey soils, respectively, whereas the maximum fresh weight was 0.675 g in loamy soil. In the same way, the average fresh weight of L. perenne in sandy soil was 0.45 g, whereas that in clayey soil was 0.705 g, with an increase of 56.7%. The average fresh weight of L. perenne in loamy soil reached the maximum value of 0.79 g, which was 12.1% higher than that in clayey soil. The average fresh weight of S. sudanense in sandy soil was 0.515 g, that in clayey soil was 0.73 g, and that in loamy soil reached the maximum value of 0.985 g. This finding indicated that loamy soil was the most suitable soil type for the growth of all three plants, followed by clayey and sandy soils. Under these six treatment tests (Table 4), the fresh weight of F. arundinacea, L. perenne, and S. sudanense did not change significantly, indicating the effects of 2000 mg L−1 of glycine and maltose on the fresh weight of the three plants had little difference.
Table 5 shows that among the three soil conditions, F. arundinacea, L. perenne, and S. sudanense achieved maximum height in loamy soil, followed by sandy and clayey soils. In sandy soil, the plant height values of F. arundinacea with the addition of these two kinds of root exudates were 35.1 and 36.3 cm, respectively (Table 5). It indicates that these root exudates had a minimal effect on plant height. Considering that plant height is affected by various factors and is very accidental, this slight difference can be ignored. Therefore, the average plant height of F. arundinacea in sandy soil was 35.7 cm. When the growth medium was changed from loamy soil to sandy soil, the average plant height of L. perenne changed from 40.25 cm to 39.35 cm (Table 5). Compared with the sharp decrease of plant height values of S. sudanense (F2,15 = 826.418, p < 0.05) and F. arundinacea (F2,15 = 1561.503, p < 0.05), the plant height of L. perenne was very stable and almost did not decrease.
Table 6 shows that plants showed their best performance in sandy soil among the three soil types. F. arundinacea, L. perenne, and S. sudanense can effectively remove total petroleum hydrocarbons with degradation rates ranging from 45.23 to 60.52% in sandy soil, followed by loamy soil. The results obtained in clayey soil were the worst. Among the two kinds of root exudates, 2,000 mg L−1 of maltose had a better total petroleum hydrocarbons removal effect than 2,000 mg L−1 of glycine (Table 6). In loamy soil, the degradation rate of F. arundinacea, L. perenne, and S. sudanense reached 31.98–52.43%, which was 0.71–0.87 times that in sandy soil. In clayey soil, the degradation rates of F. arundinacea, L. perenne, and S. sudanense ranged from 13.16 to 31.23%, which were 0.29–0.52 times lower than those obtained in sandy soil, indicating that sandy soil was suitable for the remediation of petroleum hydrocarbon-contaminated soil using these three plants. When the root exudate was changed from 2000 mg L−1 of glycine to 2000 mg L−1 of maltose (Table 6), F. arundinacea, L. perenne, and S. sudanense degradation rates of total petroleum hydrocarbons increased by 12.61% (F8,18 = 7631.501, p < 0.05), 14.47% (F8,18 = 6934.322, p < 0.05), and 19.26% (F8,18 = 14014.192, p < 0.05) in sandy soil, respectively. F. arundinacea, L. perenne, and S. sudanense performance in loamy soil was very similar to that in sandy soil, whereas clayey soil only increased by 1.59% (F8,18 = 7631.501, p > 0.05), 1.89% (F8,18 = 6934.322, p > 0.05), and 13.30% (F8,18 = 14014.192, p > 0.05), respectively. These results indicated that changing the root exudates can hardly improve the degradation rate of total petroleum hydrocarbons in clayey soil. The petroleum hydrocarbon-contaminated soil had a certain degree of natural degradation ability, but the combined remediation effect of plants with maltose was more significant.
Analysis of soil types, root exudate types, and their interaction (Table 7) showed that soil types had significant influence on fresh weight (F. arundinacea: F = 13.259, df = 2, p < 0.05; L. perenne: F = 6.825, df = 2, p < 0.05; S. sudanense: F = 6.071, df = 2, p < 0.05), plant height (F. arundinacea: F = 1631.674, df = 2, p < 0.01; L. perenne: F = 255.834, df = 2, p < 0.01; S. sudanense: F = 275.569, df = 2, p < 0.01), and degradation rate of total petroleum hydrocarbons (F. arundinacea: F = 84.405, df = 2, p < 0.01; L. perenne: F = 39.381, df = 2, p < 0.01; S. sudanense: F = 105.226, df = 2, p < 0.01) of the three plants. Root exudate types and its interaction with soil types had no significant effect on plant indicators.
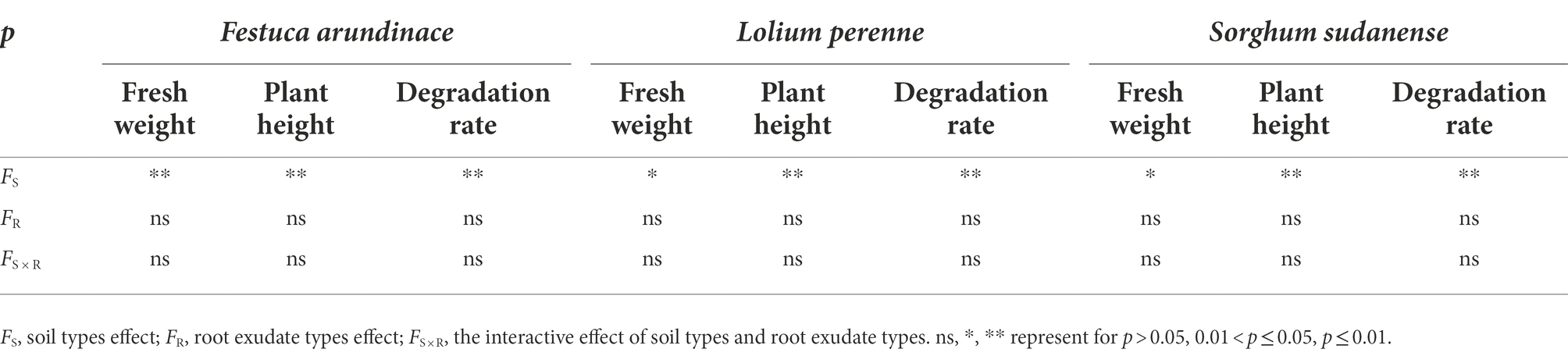
Table 7. Analysis of effects of soil types, root exudate types and their interaction on plant indicators.
Discussion
Removal effect of root exudates on cadmium under different soil conditions
Soil structure is composed of micro and macro aggregates containing solid, liquid, gas and soil organisms (Centenaro et al., 2018). Different types of soil have different structures, particle distribution, pore size distribution, water and gas storage capacity, and biological composition (Blume et al., 2016). The bulk flow movement of water and nutrients to the roots varies by soil texture (Chapman et al., 2012). The findings of this study showed that S. sudanense had higher biomass in clayey soil at different concentrations of cadmium pollution. Under the same root exudates condition, the biomass of leaves-stems of S. sudanense in the clayey soil was significantly higher than that in the sandy soil and loamy soil. It indicates that clayey soil was more suitable for plant growth than the other soil types. Clayey soil has uniform distribution of various components, good nutrient content, and strong water retention capacity; thus, it is very suitable for rapid plant growth and for achieving high biomass (Yang et al., 2013). Moreover, the accumulation of heavy metals in the soil is significantly correlated with soil particles’ surface area and pore volume. Clayey soil also has a higher granular surface area, contributing to increased retention of metals in the pore spaces (Chen et al., 2016). Sandy soil has high sand, and quartz contents, low nutrient content, poor fertility retention, and plants’ presence in sandy soils reduces porosity and pore-connectivity (Bacq-Labreui et al., 2018). Wherefore, it is difficult for plants to effectively obtain conducive nutrients for their growth during the growth period, resulting in slow growth and small biomass. As Bharti et al. (2017) reported, lack of soil structure, inadequate water supply and nutrient deficiency generally exacerbate metal toxicity in the contaminated soils. The adsorption degree of cadmium ions in the sandy soil colloid is not as good as those in loamy and clayey soils, leading to the activation of heavy metal ions and easy absorption by plants (Yang et al., 2017). In this experiment, under the same concentration of cadmium and the same root exudates, the accumulation of cadmium in plants in sandy soil were almost significantly higher than that in loamy soil and clayey soil. This maybe because of the migration resistance of sandy soil to heavy metals which is much lower than that of loamy soil and clayey soil. Wang and Lei (2018) showed that the penetration time of all studied metals in sandy soil is less, and the pore flow velocity is much higher than the pore flow velocity in other soils.
Adding low carbohydrate root exudates to soil can effectively improve the extraction of heavy metals by plants (Huang and Blaylock, 1998; Heděnec et al., 2014). Sun et al. (2009) studied the effect of citric acid on the accumulation of heavy metals in S. alfredii H. by pot experiment and found that 8 mmol kg−1 of citric acid treatment could significantly enhance the extraction efficiency of Cd and Zn by S. alfredii H. Ehsan et al. (2014) suggested that exogenous citric acid application can significantly reduce Cd toxicity in Brassica napus L. plants by increasing plant biomass, photosynthetic and growth parameters, and by reducing oxidative stress by Cd stress. The citric acid application can alleviate Cd-induced phytotoxicity in B. juncea seedlings. It is achieved by upregulating the metabolites of primary (TCA cycle) and secondary metabolism (phenolic compounds; Kaur et al., 2018). After adding three kinds of root exudates in the three soil conditions, citric acid promoted the growth of S. sudanense more significantly than glycine and maltose. This may be due to the fact that citric acid can induce plants to produce more sugar to provide energy for the body under Cd stress, thus ensuring normal cell metabolism and maintaining the function of the stress system (Dixit et al., 2001; Ghori et al., 2019). Accumulation of cadmium in plants is related not only to plant growth, but also to the degree of the intact plasma membrane. The plasma membrane can control membrane potential, the transmembrane transport of ions and solutes, and growth (Chen et al., 2020). Under the stress of Cd, the plasma membrane will be destroyed to some degree, and the addition of appropriate concentrations of root secretions can alleviate the peroxidation of the cytoplasmic membrane, thus promoting the transfer and enrichment of Cd in plants. Compared with the other two root exudates, citric acid has a better removal effect on Cd in soil, possibly because citric acid can provide more hydrogen ions, which can dissolve soil minerals to a certain extent. Moreover, citric acid has a special chemical structure and the existence of three carboxyl groups make its complexation ability to Cd stronger than glycine and maltose.
Removal effect of root exudates on total petroleum hydrocarbons under different soil conditions
Soil texture would affect the phytoremediation efficiency by controlling the bioavailability of the plant nutrients and the contaminants (Figueiredo et al., 2016). Huesemann et al. (2004) stated that both plant roots and organisms are more active, and the contaminants have weaker binding in coarser soil textures. In this study, under the same plant condition, the removal rate of petroleum hydrocarbons in the clayey soil was significantly lower than that in the sandy soil and loamy soil. Compared to sandy soils and loamy soils, the binding of molecules is stronger in clayey soils, and the presence of petroleum hydrocarbon makes them more sticky, cohesive and blocking, which accounts for the lower bioavailability of contaminants in clayey soils (Abdel-Moghny et al., 2012). According to Mohammad (2016), particle and pore size distributions significantly enhance or reduce pollutant degradation by influencing soil aeration, bulk density, hydrocarbon movement, and adsorption/desorption of pollutants. Sandy soil has good aeration and loose soil structure. Thus, the microbes in plant roots can easily obtain oxygen, energy, and quickly remove high amounts of organic pollutants in sandy soil (Albergaria et al., 2008). Furthermore, sandy soil is more permeable than other soil types; hence, some petroleum hydrocarbon may be lost in the presence of rain or when plants are watered. Clayey soil has very small crevices, and it is difficult for the root exudate solution to circulate to the surface of plant roots through the pores. Therefore, the use of different root exudates to degrade total petroleum hydrocarbons is limited.
Shoot and root traits and their relationships are affected by soil texture (Foxx and Wojcik, 2021). Plant root structure changes accordingly to maximize nutrient absorption (Giehl et al., 2014). L. perenne is drought-resistant and the root cluster can steadily extract certain nutrients from dry soil, which can survive for a long time even under extremely low water conditions (Safari et al., 2022). In this study, plant height of F. arundinacea and S. sudanense on loamy soil was significantly higher than that on sandy and clayey soil. Loamy soil produced the tallest plants due to its greater water and nutrient availability of organic matter (Sorgonà et al., 2007). Phillips et al. (2012) reported that biodegradation of petroleum hydrocarbon in loam and sandy soil is inversely proportional to pollutant concentration under appropriate soil organic matter content and good microbial degradation conditions. The aeration and water permeability in the clayey soil are poor. The particle size of the pellets and large pore space between them may reduce their moisture content (Chapman et al., 2012), which leads to the slow decomposition of organic matter. Thus, it is difficult for plants to absorb nutrients and water from the clayey soil.
Besides organic pollutants, the presence of other carbon sources in the rhizosphere can produce catabolism inhibition, and microorganisms preferentially consume carbon sources that are more easily mineralized. The addition of low molecular weight root exudates such as sugars, organic acids and amino acids can make the soil microbial community produce a rapid response in respiration (Martin et al., 2014). In our study, we have found that planting, addition of maltose and glycine can significantly facilitate the degradation of petroleum hydrocarbons in loamy soil and clayey soil. Molina et al. (2021) found that the maltose concentration in the clover root exudates with bacterial presence was significantly lower than that of the exudates without bacteria. Maltose can enhance the degradation of total petroleum hydrocarbons, probably because it can provide a large amount of energy and nutrient source for rhizosphere microorganisms, increasing the density and activity of microorganisms (Al-Ali et al., 2018) and then promoting the removal of petroleum hydrocarbon in soil. The composition and content of organic acids and amino acids in root exudates have a significant influence on the balance of adsorption and desorption of stress substances in soil (Rohrbacher and St-Arnaud, 2016). A large number of highly hydrophobic organic compounds are present in petroleum, and bioavailability is also limited due to their low activity which is firmly fixed in the soil matrix. Glycine can promote the degradation of total petroleum hydrocarbons in the soil to a certain extent, possibly because of the competitive adsorption between glycine and polar groups of organic pollutants on the surface of soil minerals. Such substances can help the desorption of hydrophobic organic matter in the soil, improve its bioavailability, thus accelerating the degradation of total petroleum hydrocarbons. In addition, soluble organic matter in root exudates can directly interact and combine with organic pollutants, which improves the mobility of organic pollutants in soil facilitating the desorption of organic pollutants.
Conclusion
Soil texture and root exudates significantly influence the effect of soil phytoremediation. Under the condition of cadmium pollution, clayey soil is more suitable for plant growth, meanwhile citric acid can promote the enrichment of cadmium in S. sudanense, F. arundinacea, and L. perenne. S. sudanense demonstrated the best total petroleum hydrocarbons degradation in sandy soil. Changing the root exudate can effectively improve the efficiency of total petroleum hydrocarbons degradation by plants in sandy soil. Perennial L. perenne has a strong ability to degrade total petroleum hydrocarbons. Therefore, L. perenne is recommended for the remediation of petroleum hydrocarbon-contaminated oil fields. In conclusion, the combined remediation technology of root exudates and plants is suitable for cadmium and petroleum contaminated soil. At the same time, soil texture should be considered a critical factor in the future application of phytoremediation technology to provide a more comprehensive idea for further improving the efficiency of soil remediation. The soil studied in this paper was artificially prepared, and there are certain differences from the natural soil in terms of pollutant types, concentration, aging degree and microbial community. Subsequent studies on actual soil remediation can be carried out to verify its effectiveness. In addition, the composition of root exudates is complex, and only organic acids, carbohydrates, and amino acids were studied in this paper. More experiments are needed to explore the effect and influence of other components on the degradation of heavy metals and petroleum in the future. This study provides a new method for remediation of soil contaminated by low and medium concentrations of cadmium and petroleum hydrocarbons. L. perenne is recommended as a priority for remediation of contaminated soil in oilfield areas.
Data availability statement
The original contributions presented in the study are included in the article/supplementary material, further inquiries can be directed to the corresponding author.
Author contributions
All authors contributed to the study conception and design. MH and CC: conceptualization, methodology, and writing of the original draft and data curation. PM and ZL: review, MH: visualization and editing. All authors contributed to the article and approved the submitted version.
Funding
This work was funded by the “13th five-year” National Major Science and Technology Project of China (grant no. 2016ZX05040-002).
Conflict of interest
The authors declare that the research was conducted in the absence of any commercial or financial relationships that could be construed as a potential conflict of interest.
Publisher’s note
All claims expressed in this article are solely those of the authors and do not necessarily represent those of their affiliated organizations, or those of the publisher, the editors and the reviewers. Any product that may be evaluated in this article, or claim that may be made by its manufacturer, is not guaranteed or endorsed by the publisher.
References
Abdel-Moghny, T. H., Mohammed, R. S. A., El-Seyed, E., Aly, S. M., and Snousy, M. G. (2012). Effect of soil texture on remediation of hydrocarbons contaminated soil at El-Minia district, upper Egypt. ISRN Chem. 2012, 1–13. doi: 10.5402/2012/406598
Al-Ali, A., Deravel, J., Krier, F., Béchet, M., Ongena, M., and Jacques, P. (2018). Biofilm formation is determinant in tomato rhizosphere colonization by bacillus velezensis FZB42. Environ. Sci. Pollut. Res. 25, 29910–29920. doi: 10.1007/s11356-017-0469-1
Albergaria, J. T., Maria da Conceição, M., and Delerue-Matos, C. (2008). Soil vapor extraction in sandy soils: influence of airflow rate. Chemosphere 73, 1557–1561. doi: 10.1016/j.chemosphere.2008.07.080
Aram, S., Weisany, W., Daliri, M. S., and Mirkalaie, S. A. A. M. (2021). Phenology, physiology, and fatty acid profile of canola (Brassica napus L.) under agronomic management practices (direct seeding and transplanting) and zinc foliar application. J. Soil Sci. Plant Nutr. 21, 1735–1744. doi: 10.1007/s42729-021-00475-3
Arshad, M., Khan, A., Hussain, I., Badar-uz-Zaman, A. M., Iqbal, M., Soja, G., et al. (2017). The reduction of chromium (VI) phytotoxicity and phytoavailability to wheat (Triticum aestivum L.) using biochar and bacteria. Appl. Soil Ecol. 114, 90–98. doi: 10.1016/j.apsoil.2017.02.021
Awa, S. H., and Hadibarata, T. (2020). Removal of heavy metals in contaminated soil by phytoremediation mechanism: a review. Water Air Soil. Pollut. 231, 1–15. doi: 10.1007/s11270-020-4426-0
Bacq-Labreui, A., Crawford, J., Mooney, S. J., Neal, A. L., Akkari, E., McAuliffe, C., et al. (2018). Effects of cropping systems upon the three-dimensional architecture of soil systems are modulated by texture. Geoderma 332, 73–83. doi: 10.1016/j.geoderma.2018.07.002
Bharti, V. S., Dotaniya, M. L., Shukla, S. P., and Yadav, V. K. (2017). “Managing soil fertility through microbes: prospects, challenges and future strategies” in Agro-Environmental Sustainability. eds. J. Singh and G. Seneviratne (Cham: Springer), 81–111.
Blume, H. P., Brümmer, G. W., Horn, R., Kandeler, E., Kögel-Knabner, I., Kretzschmar, R., et al. (2016). Scheffer/Schachtschabel: Lehrbuch der Bodenkunde. 16. Auflage 2010, 2016 (Nachdruck). Springer-Verlag Available at: https://xs.dailyheadlines.cc/books?hl=zh-CN&lr=&id=HA5QDwAAQBAJ&oi=fnd&pg=PR5&dq=10.1007/978-3-662-49960-3&ots=LWL8l0cEC4&sig=WGIVKQvNqwB1onfNVVioovuhKBk
Cao, X. R., Wang, X. Z., Lu, M., Hamid, Y., Lin, Q., Liu, X. X., et al. (2021). The cd phytoextraction potential of hyperaccumulator Sedum alfredii-oilseed rape intercropping system under different soil types and comprehensive benefits evaluation under field conditions. Environl. Pollut 285:117504. doi: 10.1016/j.envpol.2021.117504
Centenaro, G., Hudek, C., Zanella, A., and Crivellaro, A. (2018). Root-soil physical and biotic interactions with a focus on tree root systems: a review. Appl. Soil Ecol. 123, 318–327. doi: 10.1016/j.apsoil.2017.09.017
Chapman, N., Miller, A. J., and Lindsey, W. W. R. (2012). Roots, water, and nutrient acquisition: let’s get physical. Trends Plant Sci. 17, 701–710. doi: 10.1016/j.tplants.2012.08.001
Chen, Y. M., Gao, J. B., Yuan, Y. Q., Ma, J., and Yu, S. (2016). Relationship between heavy metal contents and clay mineral properties in surface sediments: implications for metal pollution assessment. Cont. Shelf Res. 124, 125–133. doi: 10.1016/j.csr.2016.06.002
Chen, C., Li, Z. B., Li, S. J., Deng, N. X., and Mei, P. (2020). Effects of root exudates on the activation and remediation of cadmium ion in contaminated soils. Environl. Sci. Pollut Res. 27, 2926–2934. doi: 10.1007/s11356-019-07263-8
Dixit, V., Pandey, V., and Shyam, R. (2001). Differential antioxidative responses to cadmium in roots and leaves of pea (Pisum sativum L. cv. azad). J. Exp. Bot. 52, 1101–1109. doi: 10.1093/jexbot/52.358.1101
Ehsan, S., Ali, S., Noureen, S., Mahmood, K., Farid, M., Ishaque, W., et al. (2014). Citric acid assisted phytoremediation of cadmium by Brassica napus L. Ecotox. Environ. Safe 106, 164–172. doi: 10.1016/j.ecoenv.2014.03.007
Estefan, G, Sommer, R, and Ryan, J. (2013). Methods of soil, plant, and water analysis: A manual for the West Asia and North Africa region, 3rd Edn.. Beirut: ICARDA, pp:65–119
Farrag, K., Senesi, N., Rovira, P. S., and Brunetti, G. (2012). Effects of selected soil properties on phytoremediation applicability for heavy-metal-contaminated soils in the Apulia region, southern Italy. Environ. Monit. Assess. 184, 6593–6606. doi: 10.1007/s10661-011-2444-5
Figueiredo, M. A., Leite, M. G. P., and Kozovits, A. R. (2016). Influence of soil texture on nutrients and potentially hazardous elements in Eremanthus erythropappus. Int. J. Phytoremediation 18, 487–493. doi: 10.1080/15226514.2015.1115961
Foxx, A. J., and Wojcik, S. T. (2021). Plasticity in response to soil texture affects the relationships between a shoot and root trait and responses vary by population. Folia Oecologica 48, 199–204. doi: 10.2478/foecol-2021-0020
Ghori, N. H., Ghori, T., Hayat, M. Q., Imadi, S. R., Gul, A., Altay, V., et al. (2019). Heavy metal stress and responses in plants. Int. J. Environ. Sci. Tech. 16, 1807–1828. doi: 10.1007/s13762-019-02215-8
Giehl, R. F. H., Gruber, B. D., and von Wirén, N. (2014). It’s time to make changes: modulation of root system architecture by nutrient signals. J. Exp. Bot. 65, 769–778. doi: 10.1093/jxb/ert421
Gómez-Sagasti, M. T., Epelde, L., Alkorta, I., and Garbisu, C. (2016). Reflections on soil contamination research from a biologist́s point of view. Appl. Soil Ecol. 105, 207–210. doi: 10.1016/j.apsoil.2016.04.004
Heděnec, P., Novotný, D., Ust'ak, S., Honzík, R., Kovářová, M., Šimáčková, H., et al. (2014). Allelopathic effect of new introduced biofuel crops on the soil biota: a comparative study. Eur. J. Soil Biol. 63, 14–20. doi: 10.1016/j.ejsobi.2014.05.002
Hodge, A., Berta, G., Doussan, C., Merchan, F., and Crespi, M. (2009). Plant root growth, architecture and function. Plant Soil 321, 153–187. doi: 10.1007/s11104-009-9929-9
Hou, J. Y., Wang, Q. L., Liu, W. X., Zhong, D. X., Ge, Y. Y., Christie, P., et al. (2021). Soil microbial community and association network shift induced by several tall fescue cultivars during the phytoremediation of a petroleum hydrocarbon-contaminated soil. Sci. Total Environ. 792:148411. doi: 10.1016/J.SCITOTENV.2021.148411
Hu, Y., Zhang, P., Yang, M., Liu, Y., Zhang, X., Feng, S., et al. (2020). Biochar is an effective amendment to remediate cd-contaminated soils—a meta-analysis. J. Soils Sediments 20, 3884–3895. doi: 10.1007/s11368-020-02726-9
Huang, J. W., and Blaylock, M. J. (1998). Phytoremediation of uranium-contaminated soil: role of organic acids in triggering uranium hyperaccumulation in plants. Environ. Sci. Technal 32, 2004–2008. doi: 10.1021/es971027u
Huesemann, M. H., Hausmann, T. S., and Fortman, T. J. (2004). Does bioavailability limit biodegradation? A comparison of hydrocarbon biodegradation and desorption rates in aged soils. Biodegradation 15, 261–274. doi: 10.1023/B:BIOD.0000042996.03551.f4
Hussain, F., Hussain, I., Khan, A. H. A., Muhammad, Y. S., Iqbal, M., Soja, G., et al. (2018). Combined application of biochar, compost, and bacterial consortia with italian ryegrass enhanced phytoremediation of petroleum hydrocarbon contaminated soil. Environ. Exp. Bot. 153, 80–88. doi: 10.1016/j.envexpbot.2018.05.012
Kaur, R., Yadav, P., Thukral, A. K., Sharma, A., Bhardwaj, R., Alyemeni, M. N., et al. (2018). Castasterone and citric acid supplementation alleviates cadmium toxicity by modifying antioxidants and organic acids in Brassica juncea. J. Plant Growth Regul. 37, 286–299. doi: 10.1007/s00344-017-9727-1
Khan, A. H. A., Kiyani, A., Mirza, C. R., Butt, T. A., Barros, R., Ali, B., et al. (2021). Ornamental plants for the phytoremediation of heavy metals: present knowledge and future perspectives. Environ. Res. 195:110780. doi: 10.1016/j.envres.2021.110780
Khan, A. H. A., Tanveer, S., Alia, S., Anees, M., Sultan, A., Iqbal, M., et al. (2017). Role of nutrients in bacterial biosurfactant production and effect of biosurfactant production on petroleum hydrocarbon biodegradation. Eco. Eng. 104, 158–164. doi: 10.1016/j.ecoleng.2017.04.023
Li, L., Li, Y., Wang, Y., Tang, M., and Ai, S. (2021). Si-rich amendment combined with irrigation management to reduce cd accumulation in brown rice. J. Soil. Sci. Plant. Nutr. 21, 3221–3231. doi: 10.1007/s42729-021-00601-1
Li, J., Wang, W., Guo, M., Kang, H., Wang, Z., Huang, J., et al. (2020). Effects of soil texture and gravel content on the infiltration and soil loss of spoil heaps under simulated rainfall. J. Soils Sediments 20, 3896–3908. doi: 10.1007/s11368-020-02729-6
Liao, Q. H., Liu, H., Lu, C., Liu, J., Waigi, M. G., and Ling, W. T. (2021). Root exudates enhance the PAH degradation and degrading gene abundance in soils. Sci. Total Environ. 764:144436. doi: 10.1016/j.scitotenv.2020.144436
Liu, X. Y., Shen, S. Y., Zhang, X. Y., Chen, X. T., Jin, R. L., and Li, X. Y. (2020b). Effect of enhancers on the phytoremediation of soils polluted by pyrene and Ni using Sudan grass (Sorghum sudanense (piper) Stapf.). Environ. Sci. Pollut. Res. 27, 41639–41646. doi: 10.1007/s11356-020-09934-3
Liu, H., Tan, X., Guo, J., Liang, X., Xie, Q., and Chen, S. (2020a). Bioremediation of oil-contaminated soil by combination of soil conditioner and microorganism. J. Soils Sediments 20, 2121–2129. doi: 10.1007/s11368-020-02591-6
Madejón, P., Domínguez, M. T., Gil-Martínez, M., Navarro-Fernández, C. M., Montiel-Rozas, M., Madejón, E., et al. (2018). Evaluation of amendment addition and tree planting as measures to remediate contaminated soils: the Guadiamar case study (SW Spain). Catena 166, 34–43. doi: 10.1016/j.catena.2018.03.016
Mao, Z., Liu, B., Fang, X., Ma, J., Liu, D., Gao, Z., et al. (2022). Effects of different fertilizer treatments on AC electric field–assisted phytoremediation efficiency of cd-contaminated soil by willow and sedum. J. Soils Sediments 22, 1460–1468. doi: 10.1007/s11368-022-03148-5
Marschner, H., Kirkby, E., and Cakmak, I. (1996). Effect of mineral nutritional status on shoot-root partitioning of photoassimilates and cycling of mineral nutrients. J. Exp. Bot. 47, 1255–1263. doi: 10.1093/jxb/47.Special_Issue.1255
Martin, B. C., George, S. J., Price, C. A., Ryan, M. H., and Tibbett, M. (2014). The role of root exuded low molecular weight organic anions in facilitating petroleum hydrocarbon degradation: current knowledge and future directions. Sci. Total Environ. 472, 642–653. doi: 10.1016/j.scitotenv.2013.11.050
Mohammad, A. H. (2016). Importance of soil physical characteristics for petroleum hydrocarbons phytoremediation: a review. Afr. J. Environ. Sci. Technol. 10, 394–405. doi: 10.1016/j.scitotenv.2013.11.050
Molina, L., Udaondo, Z., Montero-Curiel, M., Wittich, R. M., García-Puente, A., and Segura, A. (2021). Clover root exudates favor novosphingobium sp. HR1a establishment in the rhizosphere and promote phenanthrene rhizoremediation. Msphere 6, 00412–00421. doi: 10.1128/mSphere.00412-21
Nouri, H., and Hashempour, Y. (2021). Phytoremediation of cd and Pb in polluted soil: a systematic review. Int. J. Environ. Anal. Chem. 5, 1–10. doi: 10.1080/03067319.2021.1946688
Pan, H., Liang, J., Zhao, Y., and Li, F. (2020). Facing the 3rd national land survey (cultivated land quality): soil survey application for soil texture detection based on the high-definition field soil images by using perceptual hashing algorithm (pHash). J. Soils Sediments 20, 3427–3441. doi: 10.1007/s11368-020-02657-5
Parnian, A., Parnian, A., Pirasteh-Anosheh, H., Furze, J. N., Prasad, M. N. V., Race, M., et al. (2022). Full-scale bioremediation of petroleum-contaminated soils via integration of co-composting. J. Soils Sediments 22, 2209–2218. doi: 10.1007/s11368-022-03229-5
Phillips, L. A., Greer, C. W., Farrell, R. E., and Germida, J. J. (2012). Plant root exudates impact the hydrocarbon degradation potential of aweathered-hydrocarbon contaminated soil. Appl. Soil Ecol. 52, 56–64. doi: 10.1016/j.apsoil.2011.10.009
Rohrbacher, F., and St-Arnaud, M. (2016). Root exudation: the ecological driver of hydrocarbon rhizoremediation. Agronomy 6:19. doi: 10.3390/agronomy6010019
Rosa, S. D., Silva, C. A., Carletti, P., and Sawaya, A. C. H. F. (2021). Maize growth and root organic acid exudation in response to water extract of compost application. J. Soil Sci. Plant Nutr. 21, 2770–2780. doi: 10.1007/s42729-021-00564-3
Safari, S., Nazari, F., Vafaee, Y., and Teixeira Da Silva, J. A. (2022). Impact of rice husk biochar on drought stress tolerance in perennial ryegrass (Lolium perenne L.). J. Plant Growth Regul. 1–17. doi: 10.1007/s00344-022-10588-3
Sorgonà, A., Abenavoli, M. R., Gringeri, P. G., and Cacco, G. (2007). Comparing morphological plasticity of root orders in slow- and fast-growing citrus rootstocks supplied with different nitrate levels. Ann. Bot. 100, 1287–1296. doi: 10.1093/aob/mcm20710.1093/aob/mcm207
Sun, Y. B., Zhou, Q. X., An, J., Liu, W. T., and Liu, R. (2009). Chelator-enhanced phytoextraction of heavy metals from contaminated soil irrigated by industrial wastewater with the hyperaccumulator plant (Sedum alfredii Hance). Geoderma 150, 106–112. doi: 10.1016/j.geoderma.2009.01.016
Sylvain, B., Mikael, M. H., Florie, M., Emmanuel, J., Marilyne, S., Sylvain, B., et al. (2016). Phytostabilization of as, Sb and Pb by two willow species (S. viminalis and S. purpurea) on former mine technosols. Catena 136, 44–52. doi: 10.1016/j.catena.2015.07.008
Tiecher, T. L., Ceretta, C. A., Ferreira, P. A., Lourenzi, C. R., Tiecher, T., Girotto, E., et al. (2016). The potential of Zea mays L. in remediating copper and zinc contaminated soils for grapevine production. Geoderma 262, 52–61. doi: 10.1016/j.geoderma.2015.08.015
Tripathi, S., Singh, V. K., Srivastava, P., Singh, R., Devi, R. S., Kumar, A., et al. (2020b). “Phytoremediation of organic pollutants: current status and future directions,” in Abatement of Environmental Pollutants. eds. P. Singh, A. Kumar and A. Borthakur (Kidlington, United Kingdom: Elsevier).
Tripathi, S., Srivastava, P., Devi, R. S., and Bhadouria, R. (2020a). “Influence of synthetic fertilizers and pesticides on soil health and soil microbiology,” in Agrochemicals Detection, Treatment and Remediation. ed. M. N. V. Prasad (Oxford, United Kingdom: Butterworth-Heinemann), 25–54.
Wang, Y. J., Cao, Q., Chang, X. H., Wang, D. M., Wang, Y. J., Yang, Y. S., et al. (2021b). Effects of chemical regulation on wheat yield and quality under different soil conditions. Crops 2, 96–100.
Wang, Z., and Lei, G. (2018). “Study on penetration effect of heavy metal migration in different soil types,” in IOP Conference Series: Materials Science and Engineering, Vol. 394. (United Kingdom: IOP Publishing), 052033.
Wang, G. P., Xiao, B., Li, S. L., Sun, F. H., and Li, Y. B. (2021a). Characteristics of penetration resistance of moss-dominated biocrusts on aeolian and loessal soils and its influencing factors on Chinese loess plateau. Soil 53, 173–182. doi: 10.13758/j.cnki.tr.2021.01.024
Wyszkowska, J., Borowik, A., and Kucharski, J. (2019). The resistance of Lolium perenne L. × hybridum, Poa pratensis, Festuca rubra, F. arundinacea, Phleum pratense and Dactylis glomerata to soil pollution by diesel oil and petroleum. Plant Soil Environ. 65, 307–312. doi: 10.17221/42/2019-PSE
Yang, F. S., Cao, M. M., Li, H. E., Wang, X. H., and Bi, C. F. (2013). Simulation of sediment retention effects of the single seabuckthorn flexible dam in the Pisha sandstone area. Ecol. Eng. 52, 228–237. doi: 10.1016/j.ecoleng.2012.11.010
Yang, W. T., Zhou, H., Gu, J. F., Liao, B. H., Peng, P. Q., and Zeng, Q. R. (2017). Effects of a combined amendment on Pb, cd, and as availability and accumulation in rice planted in contaminated paddy soil. Soil Sediment Conta Int. J. 26, 70–83. doi: 10.1080/15320383.2017.1235132
Zhang, B. F., Zhang, L., and Zhang, X. X. (2019). Bioremediation of petroleum hydrocarbon-contaminated soil by petroleum-degrading bacteria immobilized on biochar. RSC Adv. 9, 35304–35311. doi: 10.1039/C9RA06726D
Keywords: soil, root exudates, cadmium, hydrocarbons, phytoremediation
Citation: He M, Li Z, Chen C and Mei P (2022) Impact of soil types and root exudates on cadmium and petroleum hydrocarbon phytoremediation by Sorghum sudanense, Festuca arundinace, and Lolium perenne. Front. Ecol. Evol. 10:1036765. doi: 10.3389/fevo.2022.1036765
Edited by:
Adam Cross, Curtin University, AustraliaReviewed by:
Rahul Bhadouria, University of Delhi, IndiaArtur Banach, The John Paul II Catholic University of Lublin, Poland
Copyright © 2022 He, Li, Chen and Mei. This is an open-access article distributed under the terms of the Creative Commons Attribution License (CC BY). The use, distribution or reproduction in other forums is permitted, provided the original author(s) and the copyright owner(s) are credited and that the original publication in this journal is cited, in accordance with accepted academic practice. No use, distribution or reproduction is permitted which does not comply with these terms.
*Correspondence: Ping Mei, meipinghb@126.com