Synergy of photocatalysis and fuel cells: A chronological review on efficient designs, potential materials and emerging applications
- 1Crystal Growth Centre, Anna University, Chennai, Tamil Nadu, India
- 2Institute of Physical Chemistry, Polish Academy of Sciences, Warsaw, Poland
The rising demand of energy and lack of clean water are two major concerns of modern world. Renewable energy sources are the only way out in order to provide energy in a sustainable manner for the ever-increasing demands of the society. A renewable energy source which can also provide clean water will be of immense interest and that is where Photocatalytic Fuel Cells (PFCs) exactly fit in. PFCs hold the ability to produce electric power with simultaneous photocatalytic degradation of pollutants on exposure to light. Different strategies, including conventional Photoelectrochemical cell design, have been technically upgraded to exploit the advantage of PFCs and to widen their applicability. Parallel to the research on design, researchers have put an immense effort into developing materials/composites for electrodes and their unique properties. The efficient strategies and potential materials have opened up a new horizon of applications for PFCs. Recent research reports reveal this persistently broadening arena which includes hydrogen and hydrogen peroxide generation, carbon dioxide and heavy metal reduction and even sensor applications. The review reported here consolidates all the aspects of various design strategies, materials and applications of PFCs. The review provides an overall understanding of PFC systems, which possess the potential to be a marvellous renewable source of energy with a handful of simultaneous applications. The review is a read to the scientific community and early researchers interested in working on PFC systems.
1 Introduction
The recent idea to explore renewable power sources has been crucial due to many vital environmental concerns of pollution and depletion of natural resources. Researchers have already understood the need for efficient mechanisms to restore the environment and to produce sustainable energy. In this context, photocatalysis has shown a tremendous impact with its compatibility to produce energy in the form of hydrogen fuel and to simultaneously degrade a wide variety of pollutants from polluted water sources. Consumption of natural solar irradiation and simplicity in design makes photocatalysis a promising candidate in arenas of green energy production and wastewater treatment (Roberta L and Wilson F, 2001; Mao et al., 2012; Zhao et al., 2015; Puga, 2016; Ayekoe et al., 2017; Miseki and Sayama, 2019).
Photocatalysis is a synergistic combination of photochemistry and catalysis (Saravanan et al., 2017). In this method, light energy and catalytic property are simultaneously used to enhance the rate of a reaction. Akira Fujishima and Honda pioneered an experiment on the photocatalytic splitting of water using TiO2 in 1972, which remains as the foundation work on photocatalysis (FUJISHIMA and HONDA, 1972). Subsequently, scientific fields have witnessed a steep leap on this frontier owing to its wide range of applications in hydrogen generation, air purification, carbon dioxide reduction, antibacterial activity and wastewater treatment. (Li and Li, 2001; Dong et al., 2014; Leyland et al., 2016; Kadam et al., 2019; Wang et al., 2020c). Photocatalytic process is garnering more attention on the grounds of wastewater treatment with its capability towards complete mineralization of the pollutant at room temperature and pressure (Malato et al., 1999; Rizzo et al., 2009; Bernabeu et al., 2011). The capability of photocatalysis to perform wasteless degradations and superior response towards natural solar irradiation unceasingly drives researchers to search for new horizons. Photocatalytic fuel cell (PFC), an improvised design of Photoelectrochemical cells (PECs), can be considered as a recent milestone along the way.
PECs are divided into three categories: Regenerative Solar Cells, Photosynthetic Cells and Photocatalytic Fuel Cells. O’Reagen and Gratezel hold the prime report for the regenerative solar cells, where the solar energy is converted into electrical energy (O’Regan and Grätzel, 1991). In photosynthetic cells, solar energy is transformed into solar fuels, mainly hydrogen. The above-mentioned work by Fujishima and Honda was the first report in photosynthetic cells, where hydrogen evolution reaction was steered through photocatalytic water splitting (FUJISHIMA and HONDA, 1972). The photocatalytic fuel cell (PFC), evolving from photoelectrochemical (PEC) cells, is a more promising technology for organic waste remediation, electricity generation, hydrogen production, CO2 reduction, H2O2 generation, sensor applications, heavy metal reduction etc. Even though the PEC and PFC share similarities on the grounds of design and mechanism, the functional capability of PFC to extract chemical energy from organic molecules, without external bias is its key strength over PEC. In PFC, photocatalytic activity leads to electricity generation with or without hydrogen evolution, using organics as a fuel. Even though there is no specific report which can be stated as the primary report on PFC due to its prolonged transformation from PEC, Kaneko et al. was the first group to coin the term Photo Fuel cells. The reported photo fuel cell used TiO2 and Pt as photoanode and dark cathode respectively and NH3 as pollutant or “fuel” for the cell (Kaneko et al., 2005). This work kick-started studies on PFC in 2005, and from then, numerous reports have been published worldwide. Appropriate studies on PFC systems with enhanced efficiency shall be considered as a flawless scenario to tackle the upcoming global issues of water pollution and energy scarcity.
Photocatalytic fuel cell (PFC) further carries forward the concept of photocatalysis by degradation of pollutants and simultaneous electricity generation, in some cases, along with hydrogen generation (Liu et al., 2011a; Seger et al., 2012; Li J. et al., 2013; Wu et al., 2015). PFCs provide a synergistic effect of complete mineralization of pollutants by photocatalysis and sustainable electricity generation mechanism of fuel cells. In spite of various modifications constantly coming up in the design and materials of PFC, the basic strategy and principle remains intact. The basic design of a PFC consists of electrodes and suitable electrolyte as in PEC, with a substrate to be used as fuel. The photoanode is a photocatalyst semiconductor and the dark cathode is an electrocatalyst of noble metal. The electrolyte is chosen based on its ionic characteristics and the electrodes. Fuel or substrate has a wide range to choose from, since the photocatalytic property has a non-selective nature towards degrading the organics (Ueno et al., 2009). When the photoanode is composed of an n-type photocatalytic semiconductor, it acts as a negative electrode since the Fermi level lies near the conduction band edge of the n-type semiconductor. The chemical reactions occurring on the cathode purely rely on the aeration provided in the cathode compartment. In the absence of oxygen, the cathode behaves as a hydrogen electrode, whose potential is 0 V (vs. reversible hydrogen electrode) and the hydrogen evolution reactions occur (Daneshvar et al., 2004). But in the presence of oxygen, it acts as an oxygen electrode, whose potential under acidic conditions is determined by the reactions:
When the light is irradiated on the photocatalyst in the anode section, photoexcited electron-hole pairs are generated. Due to the difference in the potential levels of anode and cathode, the generated voltage drives the electrons in the external circuit, producing electrical energy. Photogenerated holes in the anode compartment work on the degradation of fuel through the conversion of hydroxyl ions to hydroxyl radical, which is one of the most reactive oxygen species. The photogenerated electrons contribute to hydrogen evolution reactions at the cathode in the absence of oxygen. Ion transport membranes are used between the anode and cathode compartments to enhance the sustainability of the cell. Although this is a basic design of PFC, numerous alterations and studies have been done to improve the efficiency of the system. The plausible reactions at anodic and cathodic sites are given in Eqs 3-16, as follows:
At anode:
At cathode:
The present review article concentrates on the wide spectrum of research reports available on PFC to help the scientific community to get detailed information on an interesting and important subject from the viewpoint of renewable energy. The first review article, published by Panagiotis Lianos in 2011, discussed the basic concepts of PFC and 6 years later, the group came up with a consolidated work dealing with designs of various PFC systems (Lianos, 2011, 2017). Advanced materials used as PFC photo-electrodes and their engineering techniques were thoroughly studied by Li et al., in 2019 (Li M. et al., 2019). Ermete Antolini presented a review on PFCs in 2019, which gives a collective study on various materials and properties of PFC (Antolini, 2019). The various materials for electrodes in a PFC and the application of Fenton reactions in PFC systems were combined and presented by Yasser et al. (Vasseghian et al., 2020). A detailed review on the PFC systems focusing on their structural insights, charge transport, thermodynamic behavior and challenges were presented by Priyanka et al. in their recent review article (Mishra et al., 2021). Yun He et al. recently reviewed the PFC research, where the fundamentals and technological advancements along with various cell configurations have been thoroughly discussed (He et al., 2022). Since researchers have merged peer reviewed reports on the basics, designs, materials and properties of PFC systems, it seems to be a perfect phase to shed light on less explored, yet booming applications of PFC. A detailed review containing all the possible information along with the applications of PFC should be a higher priority at present.
2 Developments in design of PFC
When compared to PECs, a PFC differs in the biasing provided in the system to have a sustainable photo-generated charge transfer. In PECs, external electric biasing is provided for efficient transfer of photo-generated charges either with a standard electric cell or using a PV system. The need for external biasing for competent performance in PECs makes them less preferable on system design, cost and energy efficiency. PFCs do not require a peripheral biasing because either one or both electrodes are capable of photo-induced charge generation and their suitable Fermi level difference provides the required biasing. The scavenging of holes by fuel enhances the charge separation and transfer in photoelectrodes. The synergistic effects of this particular concept have been applied in many designs throughout the years.
2.1 Single chamber single photo-electrode
In this system, a photocatalytic semiconductor material and an electrocatalytic noble metal are employed as photo-anode and dark cathode, respectively, in a single chamber filled with electrolyte and fuel (Figure 1). The first ever reported photo fuel cell by Kaneko et al. followed this design, utilizing film electrodes (Kaneko et al., 2005). In most reports, n-type photoanode is used together with dark cathode to establish a steady current flow (Ueno et al., 2009; Li K. et al., 2013; Lee et al., 2016a; Xie and Ouyang, 2017). TiO2 photoanode and Pt dark cathode is the maximum reported combination in SCSP systems, owing to the higher efficiency and superior photocatalytic performance of TiO2(Antoniadou et al., 2010; Li N. et al., 2018; Xie et al., 2019). The SCSP system gained a lot of attention with its minimalism in design and simple but effective overall reactions. In this system, the required bias for the efficient separation and transfer of charge carriers is primarily constituted by the band edge positions and type of photoanode. The influence of pH of the solution in the system is curtailed in this strategy. SCSP design also benefits in reducing the distance between anode and cathode, thus dropping the total resistance of the system. The cost-effectiveness of the overall design is commendable in SCSP since the design is free from ion-exchange membranes as seen in dual chamber systems.
The photoanode is irradiated with a light source which promotes the generation of electron-hole pairs. Later, these electron-hole pairs are separated due to the difference in Fermi level energy between electrodes. The electrons move towards the dark cathode through the external circuit, which renders useful electric power. At the same time, the holes attack the organic pollutants and degrade them to low pollution causing intermediates or non-toxic byproducts.
2.2 Single chamber dual photo-electrode
In SCSP design, the use of expensive noble-metal dark cathode is a significant disadvantage. Pt is widely used as a dark cathode because of its superior activity along with its non-toxic and non-corrosive nature (Zeng et al., 2018b; Li N. et al., 2019; Ong et al., 2019; Tang et al., 2019). SCDP design comprises a photoanode and photocathode in a single chamber containing electrolyte and substrate (Figure 1). It is capable of eliminating the convention of an expensive noble-metal cathode in SCSP systems. It also simplifies the system by eliminating the use of proton exchange membranes. Dual photoelectrodes help in trapping a wide spectrum of light irradiation and synergistically induce the degradation of pollutants along with electricity generation. The photoanode should be an n-type photoelectrode, which has a valence band with positive potential capable of pollutant/fuel oxidation and the photocathode can be a p-type photoelectrode, which has a conduction band with negative potential, for oxidant reduction. Moreover, the Fermi level of the photoanode should be at a higher potential than that of the photocathode for spontaneous current flow (Chen et al., 2012). Therefore, careful choice of n-type and p-type material can induce an internal bias between the photoelectrodes which can drive the electrons from anode to cathode. SCDP provides a single chamber setup for efficient utilization of photogenerated holes at photoanode and electrons at photocathode (Nahyoon et al., 2019a; Rabé et al., 2019b).
2.3 Dual chamber single photo-electrode
In DCSP design, we have two distinct compartments, containing the same or different electrolytes, fuel in the photoanode compartment and oxidizer in the dark cathode compartment. In SCSP design, the internal biasing of the system was established by the photoelectrode and their respective band edge positions. In the DCSP system, the biasing depends on the photoelectrode and also the pH in both compartments. Even though DCSP design is more complicated and expensive involving the usage of proton exchange membrane, the wide range of combinations on photoelectrodes, electrolytes and substrates makes it a potential design to achieve proficient efficiency. The first report on DCSP designed cell for electricity generation and simultaneous hydrogen production utilizing ethanol, was by Maria Antoniadou in 2008 (Antoniadou et al., 2008). Among the dual chamber designs, the H-shaped reactor is the most widely used design (Figure 2). In DCSP, the light is irradiated on the anode site alone since it is the only photoactive electrode. The DCSP design has been effectively employed in electricity generation and simultaneous wastewater treatment. It was also recently employed for simultaneous dye degradation at the anode site and heavy metal removal at the cathode site, which hints towards the wide arena of applications this design can achieve. A wide variety of organic pollutants such as pharmaceutical drugs and dyes have been used as fuel to generate electricity using this design (Hu et al., 2015; Deng et al., 2018; Khalik et al., 2018; Pan et al., 2019).
2.4 Dual chamber dual photoelectrode system
In DCDP systems, the pros of employing dual photoelectrodes and advantages of dual chamber systems has been merged for better performance (Figure 2). The dual photoelectrodes can enhance the output voltage which will be the difference between quasi-Fermi levels of photoanode and photocathode. In this case, the electrons and holes generated in anode site will be utilized for electricity generation and degradation reaction, respectively. The photogenerated electrons of cathode side could react with oxygen to induce the oxygen reduction reaction (ORR), and photogenerated holes could be used to consume the electrons in the external circuit. Recently a formaldehyde PFC system was reported by Yanming Wang et al. where they have employed TiO2 nanorods (NRs) array as photoanode and Cu2O photocathode for formaldehyde oxidation. The micro-nano structure of the electrodes are controlled for high mobility of charge carriers, which resulted in a short-circuit current of 1.2 mA cm−2 and an open-circuit voltage of 0.58 V (Wang et al., 2020b). An improved short-circuit current density (Jsc), the open-circuit voltage (Voc) and the maximum power output (Pmax) of 5.18 mA cm−2, 0.82 V and 0.91 mW cm−2 respectively was reported by BingzhiQian et al. The group designed a DCDP system as a methanol-based PFC which incorporated ZnO/BiVO4 photoanode and Cu2O photocathode. The interior potential difference between n-type ZnO/BiVO4 and p-type Cu2O accounts for the enhanced performance by actuating the electrons on the photoanode to transfer through the external circuit to photocathode, combining with the holes and generating electricity (Qian et al., 2020). Recently a visible-light-driven photocatalytic fuel cell (PFC) with DCDP design was employed, in which graphitic carbon nitride (g-C3N4) on W/WO3 nanorod arrays (W/WNR/g-C3N4) was used as the photoanode and Fe3+-doped CuBi2O4 thin film on indium tin oxide (ITO) conductive glass (ITO/CBFeO) was used as the photocathode. The one-dimensional structure of photoanode and the Fermi level mismatch between electrodes improved the generation and transfer of charge carriers. The short circuit current and maximum power density could reach 620 μA cm−2 and 110 μW cm−2, respectively (Huang et al., 2022).
2.5 Triple chamber system
Several improvements have been brought in PFC designs to arrive at a stable system with improved efficiency. To enhance the charge separation in photoanode, many techniques have been employed in the system, such as engineering on electrode materials, anodic biasing etc. Providing a bias voltage at the anode site resulted in an improved degradation of organics, but a poor performance on the electricity generation. Sui et al., in 2015 came up with a triple chamber system, which makes use of salinity gradient power (SGP) to enhance the charge separation (Figure 3). The revocable mixing of solutions with high and low salt concentrations produces SGP (Veerman et al., 2010; Tedesco et al., 2015).
Usually, the degradation of the fuel reduces the competent charge separation and hence the current. But the triple chamber system using SGP was able to produce a stable current for a long time. The ability of chloride ions to get oxidized to chlorate or perchlorate by holes helped the salt solution to play the role of a substitute fuel which accounted for the stable current in the system (Sui et al., 2015).
2.6 Micro fuel cells
The relatively large distance between electrodes in PFC designs increases the mass transfer resistance and hence accounts for a decline in the efficiency of the whole system. A membrane between the chambers for ion transport, which facilitates the continuity of the system, was used in PFCs, which boosted the cost of conventional designs. Another major drawback is that the specific reaction surface is small, which limits cell performance. The design of PFCs always aimed at overcoming these drawbacks. The first such attempt was made by Li et al., in 2014 in the form of Optofluidic Micro Fuel Cells (Li et al., 2014).
Optofluidics provide the synergistic advantages of optics and microfluidics, such as fine flow control, enhanced surface to volume ratio and uniform light distribution (Psaltis et al., 2006; Erickson et al., 2011). Optofluidic-based design also helps in the reduction of distance between the electrodes and elimination of membranes. The first optofluidic PFC proposed by Li et al. had a stacked arrangement of electrodes and employed TiO2 and Pt as photoanode and cathode, respectively (Li et al., 2014). A modification of this design was proposed in 2016 by implementing a shoulder-to-shoulder arrangement of electrodes where the electrode materials remained intact. Uniform utilization of light irradiation was coined as a major advantage for this design (Xia M. et al., 2016). Chen et al., in 2018 took the MFC strategy to the next level by using a visible light responsive photoanode without any change in the basic design (Chen et al., 2018). Recently Liu et al. have reported an MFC which employed a photoanode and photocathode with a similar design as reported by Chen et al. Dual photoelectrode MFC have advantages of both MFCs and dual photoelectrode systems as we have explained above (Liu J. et al., 2019). Dual photoelectrode optofluidic fuel cell in which the usage of precious metal Pt electrode is avoided with a photocathode has been recently reported by Yun He et al. The cell consisted of Cu2O photocathode and a BiVO4 photoanode placed shoulder-to-shoulder in a microchamber, offered a large contact area between electrolyte and photoelectrodes. The photoelectricity conversion in using glucose resulted in open-circuit voltage and short-circuit photocurrent of 0.463 V and 0.113 mA cm−2, respectively (He et al., 2021).
2.7 Rotating disc PFC
Rotating disc reactor design has been proposed by many groups as an improved strategy among photoelectrochemical cells. Rotating disc design renders a synergistic attribute of highly efficient thin-film PEC design (in which the upper half of the rotating disk photoanode with a thin layer of pollutant solution is exposed to light radiation in air) and the conventional PEC design (in which the other half of the disk is submerged in bulk pollutant solution), irradiated by the same light source. The schematic design of the RDPFC system can be depicted as in Figure 2 with a modification of photoanode with rotating disc photoanode. The quantum yield of photogenerated charge carriers in the thin film half of the rotating disc design will be high due to enhanced interaction with light radiation. Hence there will be efficient oxidation of organic compounds. This thin film is unceasingly restored by the rotatory motion of the electrode, which also facilitates the mass transfer of pollutants and degradation products. Combining this thin film design with conventional bulk reactor design rotating disc design opens up a wider arena of innovative design (Xu et al., 2008, 2009).
Rotating disc design was adopted into photo fuel cells by Tang et al., in 2014, where TiO2 rotating disc electrode was utilized to degrade Rhodamine B(Rh B) and reactive brilliant red X-3B (Tang et al., 2014). It was shown that the adaptation of RD design decupled the hydrogen generation rate and electric flow compared with conventional design. A polypyrrole-based cathode was established on RD design by Li et al., in 2016 (Li K. et al., 2016). Later the RD design was further modified by Jun Zhang et al., in 2019 as they incorporated multiple rotating disc cathodes in PFC. In the multi cathode RD design, they succeeded in achieving a Pmax of 0.22 mW, which was 53% greater than a single cathode design. The obtained current, power and degradation efficiency values of RDPFCs reveal that the future of this design is appealing, even though the implementation is a bit complicated (Zhang et al., 2019c; 2019b).
A thin-film rotating disk photocatalytic fuel cell (PFC) reactor employed for enhanced photocatalytic reduction of CO2 to CH4. The yield of CH4 in this system was measured to be 7.62 μmol g−1 h−1. Diffusion of CO2 through the thin film and high solubility of CO2 in electrolyte enabled effective utilization of CO2 and improved conversion efficacy (Zhang et al., 2020c). A recent report was published by the same group on the gaseous formaldehyde removal using a RDPFC design with TiO2 photoanode and Pt cathode. Rotating disc design improved the efficiency of the system with its advantages of greater absorption, fast oxidation and humidity less sensitivity (Zhang et al., 2020d).
Numerous parameters affect the overall efficiency of a PFC system. Even though the efficacy of the PFC design is the major factor to look for, overall cost, simplicity, scalability and application are the other entities which play vital role. Table 1 provides a summary of the pros and cons of various designs.
2.8 Hybrid systems and novel designs
Recent progress on PFC design includes exploring the combination of conventional PFC designs with other well-explored systems and have been discussed here under the hybrid category. Hybrid systems are expected to enhance the efficiency of a single system in order to widen the applicability of the design. The first reported hybrid system combined the conventional PFC design with a Fenton reactor to efficiently degrade Reactive Black five and simultaneously produce electricity. The design incorporated an SCSP PFC design with RB5 dye and a Fenton reactor to produce better degradation efficiency and current generation than these systems separately (Nordin et al., 2017). PFC was used as a potential source for efficient working of PEC in the hybrid system proposed by Liu et al. In this PFC-PEC hybrid setup, the chemical energy of glucose is converted into electrical energy by an SCSP PFC and this electrical energy was utilized as a potential bias for the PEC reactor. TiO2 and Pt were employed as photoelectrodes in both cells, as shown in Figure 4. The synergy of the hybrid system produced an increased photocurrent and a commendable hydrogen evolution rate (Liu et al., 2017). A photovoltaic system pooled with PFC design was introduced by Zeng et al., where the group has demonstrated a self-sustaining monolithic photoelectrocatalytic/photovoltaic system (SMPP system). In this SMPP system, the photoanode comprising of WO3/BiVO4 was attached with a Si-PVC, which enhanced the absorption wavelength range of the system to produce a power density of 1,112 μW cm−2, 14 times greater than the conventional PFC reported back then.
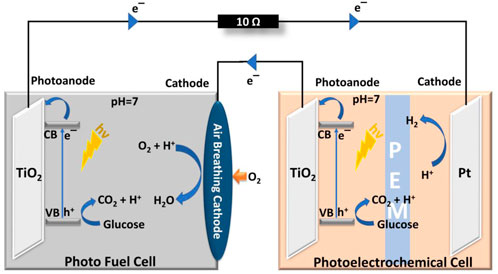
FIGURE 4. Hybrid system incorporating PFC and PEC. Reproduced with permission (Liu et al., 2017), Copyright 2022, Elsevier.
The group have recently constructed an unassisted, hybrid tandem photocatalytic fuel cell (HTPFC) constructed by adhering a silicon solar cell (SSC) to the back of a highly-active silicon-doped TiO2 nanorod array (STNR) for efficient solar hydrogen production (28.8 μmol h−1 cm−2) coupled with tetracycline degradation (Zeng et al., 2018b) (Zeng et al., 2020). If the above hybrid systems focused on enhancing the efficiency of separate systems by coupling them, some systems focused on widening the applicability of the same. A PFC-PVC system with a Pd-Cu modified Ni foam cathode was capable of removing total organic carbon (TOC) and total nitrogen from wastewater with simultaneous electricity generation (Zhang Y. et al., 2018).
Jiao et al. reported an innovative combination of CO2 photoreduction system with PFC successfully generating electricity. The 3D TiO2-Ni foam was used to reduce CO2 into hydrocarbons and this was fed into PFC as a fuel to produce electric power (Jiao et al., 2019). A step further on the design was achieved by Meijia Qiu et al. They reported a PFC combined with an Asymmetric Micro supercapacitor (AMSC), which can not only generate electric power simultaneously with wastewater degradation but also can store energy to power small portable electronics (Figure 5). TiO2 was employed as photoelectrode, Ag as counter electrode and urea as fuel to achieve a maximum power density of 3.04 μW cm−2 (Qiu et al., 2019). Another interesting hybrid system was reported recently by Peng Xu et al., where the PFC system was combined with Reverse Electro-Dialysis (RED). The stacked arrangement of cation exchange membranes (CEM) and anion exchange membranes (AEM) provides a salinity gradient to drive the RED process for wastewater treatment. The group has reported an H2O2 production and obtained a maximum power of 76 W m−2 (Xu et al., 2019b; 2019c; Xu and Xu, 2019). A visible light active PFC integrating electro-Fenton (EF) process has been recently reported employing rGO/BiO1−xI photoanode and biomass-derived N-doped carbon (BNC) cathode. The system has a maximum power density (Pmax) of 17.50 μW cm−2 with formic acid as fuel (Hu et al., 2020). A similar PFC-EF hybrid system constructed with ZnO/C photoanode and carbon cathode for enhanced power generation and Amaranth dye degradation by Shen Hui Thor et al. (Thor et al., 2020). The effect of aeration and pH on the overall efficiency of the PFC-EF system has been thoroughly studied. Dual chamber setup is employed in which, one chamber consists of PFC and the later contains the electrodes for electro Fenton reaction. The system attained a maximum power density of 2.221 μWcm−2 and maximum current density of 0.012 mAcm−2. Nordin et al. developed a hybrid electrochemical system of photocatalytic fuel cell - peroxi-coagulation (PFC-PC)which involve enhanced hydroxyl radical formation for simultaneous degradation of organic pollutant and electricity generation (Nordin et al., 2020). Furthermore, a novel design of external loop airlift photocatalytic fuel cell (APFC) has been reported by Ammar et al. The cell contains Fe@CdS and g-C3N4 immobilized on a polystyrene film as photoanode and rGO/carbon brush was used as aerated cathode. The design consists of a riser section and downcomer section. In riser section air pumping is provided for the pollutant to rise up and through the photocathode brush. Photoanode is placed as the walls of downcomer tube. The APFC design rendered maximum power density (Pmax), open circuit voltage (Voc) and short circuit current density (Jsc) were respectively 1.57 mW cm−2, 1.19 V and 2.525 mA cm−2 along with phenol degradation (Ammar et al., 2020). All of the above systems which utilizes or intend to utilize natural solar irradiation faces the major challenge of rendering continuous output throughout the day. A recent report which intends to tackle this issue is published by Zhang et al., which depicts a hybrid PFC system. The group developed a green supercapacitor assisted PFC system with a chemical bias, which aimed for storing photoelectrons by supercapacitors under light irradiation and discharging for hydrogen production under dark to enable continuous hydrogen production. The report put forward an alternative approach to achieve continuous H2 production, electricity generation and waste degradation using a supercapacitor coupled PFC system (Zhang et al., 2021).
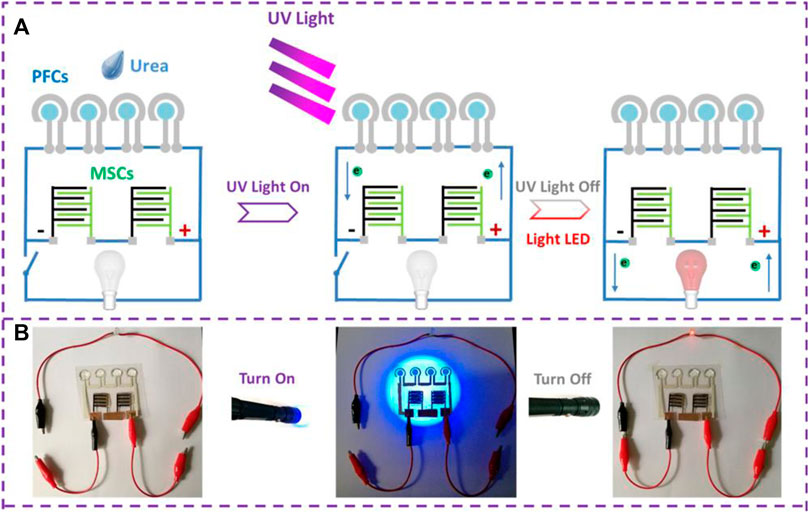
FIGURE 5. (A) Schematic diagram of self-powered system including in-plane PFCs and MSCs for powering red LED (B) real time photographs depicting four tandem planar PFCs charging two Micro Supercapacitors in series, which later power a red LED. Reproduced with permission (Qiu et al., 2019), Copyright 2019, American Chemical Society.
The hybrid systems reported so far broadly open up the doors to an incredible future of PFC and its numerous applications.
3 Advancements in materials
Materials play a vital role in almost all scientific experimental research areas. The progress in materials science has already revealed the importance of proper choice and engineering of materials based on their property. As in every other field, research based on PFCs took a great leap forward by exploring the structure-properties correlation of various materials.
3.1 Materials for anode
The photoanode of a PFC determines the power generation and degradation of organic pollutants or fuels. Hence, the development of an efficient anode material greatly determines the overall efficiency of the PFC system. In spite of extensive exploration in this field, TiO2 still remains the champion material and has been reported as a photoanode in about 70% of the total reports on PFC. Chemical inertness over wide environments, ease of synthesizing, efficient thin film deposition, availability at low-cost and non-toxic nature holds TiO2 in the prime position as a photoanode (Kaneko et al., 2005; Balis et al., 2012; Seger et al., 2012; Ogura et al., 2014; Xia M. et al., 2016; Jiao et al., 2019). However, the wide bandgap of TiO2 allows it to be active only in the ultraviolet region of the photo-spectrum and it exhibits a higher electron-hole recombination rate. To overcome these drawbacks, various approaches, such as metallic and non-metallic doping, coupling with low bandgap semiconductors and morphological variations, have been explored. All anode materials reported other than TiO2 have been presented in Table 2.
Degradation of organic substances facilitating electricity generation with CdS functionalized TiO2 was reported by Antoniadou et al., in 2009. This report can be considered as the first report on visible light activated PFC and was successfully accomplished by CdS functionalization (Antoniadou and Lianos, 2009). Heterostructured sulfide-TiO2 photoanodes have also been explored to enhance the absorption spectrum and to enhance the electron-hole separation. Many narrow bandgap semiconductors (other than sulfides) with energy band edge positions compatible with TiO2 have also been widely explored for fabricating a photoanode for PFC(Sfaelou et al., 2012; Wang et al., 2014; Liao et al., 2015; He et al., 2018; Kee et al., 2018; Raptis et al., 2018; Liu X. H. et al., 2019). Cu2O functionalized TiO2 was fabricated to enhance the current density and power density, as reported by Liu et al. (Liu et al., 2011a). Heterostructure photoanode, WO3-TiO2, was also proposed by some groups to enhance the overall efficiency of the PFC system by absorbing more photons and reducing the recombination rate. In a report by Zeng et al., TiO2 has been employed as a coating onto WO3 to overcome its drawbacks, such as sluggish charge transfer, gradual loss of photoactivity due to the formation of peroxo-species on its surface as well as rapid charge recombination due to surface defects (Hill and Choi, 2012; Kim et al., 2013; Yang et al., 2014; Zeng et al., 2018a). BiVO4-TiO2 heterostructures and ZnFe2O4-TiO2 photoanodes have also been explored to enhance light absorption, obtain maximum electron-hole separation and synergistically achieve stability (Bai et al., 2016; Xie et al., 2017; Lu et al., 2019).
Morphological variants of many materials have shown improved properties compared with their pristine powder or bulk form. As we observe down the timeline of PFC photoanode development, we can see a majority of works concentrated on one-dimensional structures of materials like nanotubes, nanorods, nanowires, etc. One-dimensional structured photoanodes with increased surface area and reduced internal resistance possess many advantages like faster electron transport rate, enhanced photon absorption rate and improved organic pollutant adsorption. In this regard, besides TiO2, which has been well explored for its one-dimensional structures, ZnO-based nanostructures have also been studied. (Liu et al., 2011b; Li J. et al., 2013; Li et al., 2016 X.; Ying et al., 2016; Zhang et al., 2019b) (Liu et al., 2011a; Li J. et al., 2013; Liao et al., 2015). Leaves-like CdS with a surface decorated by Pt-Cox nanoparticles is employed as a photoanode to improve the photocatalytic hydrogen generation. The morphological variant showed improved H2 generation (45.09 mmol h−1g−1) compared to the particles (Jia et al., 2020). The effect of co-exposed TiO2’s (001) and (101) facets on the performance of TiO2/BiVO4 photoanodes in the PFC system has been successfully studied recently (Figure 6). Nano spindles, nano cubes, nano octahedra, and nano truncated octahedra of TiO2 has been synthesized to achieve the exposure of (001) and (101) facets. The active facet morphology of TiO2 (nano spindles and nano truncated octahedra) and BiVO4 is combined to form type-II heterojunction, which enhanced the overall photocatalytic efficacy (Khalil et al., 2021).
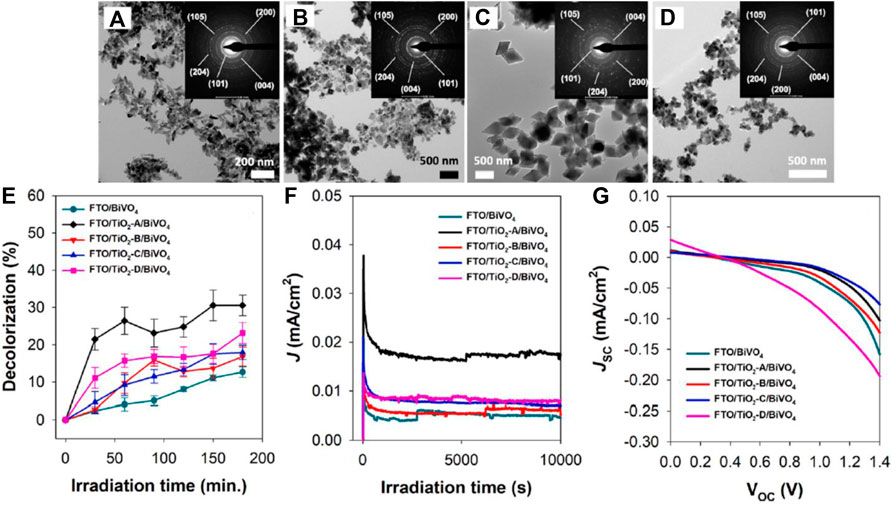
FIGURE 6. (A–D) TEM images (inset: SAED) of the as-prepared TiO2 nano spindles (TiO2-A), nano cubes (TiO2-B), nano-octahedra (TiO2-C), and nano-truncated octahedra (TiO2-D), respectively. (E–G) Performance of the PFC system in decolorization of RhB; J-t curve (measured at 0.8 V vs NHE), and JSC-VOC curve of the PFC system in the presence of RhB and Na2SO4 using different types of photoanodes under light irradiation. Reproduced with permission (Khalil et al., 2021), Copyright 2021, Elsevier.
BiOCl/Ti, BiOCl facet modified anode candidates have been proposed and showed impressive results (Li K. et al., 2013). Detailed review on BiOCl facet engineered photoanode is shown in the review by Li et al., in 2019 (Li M. et al., 2019). Nb2O5, which has a similar bandgap, but a more negative conduction band edge than TiO2, has been compared with TiO2 and WO3 in work by Hu et al. TiO2 with its impressive internal quantum yield outperformed Nb2O5 in this work (Hu et al., 2015). ZnO, which has a similar bandgap and similar bandedge positions as TiO2, has been an attractive material for photoanode fabrication with its remarkable photoactivity. However, the vulnerable nature towards oxidation limits its application (Lee et al., 2016b, 2017; Khalik et al., 2016; Kee et al., 2018; Lam et al., 2019; Ong et al., 2019). As a medium bandgap semiconductor, many have worked on BiVO4, which has been utilized as a visible light active photoanode. BiVO4 has also been combined with other metal oxide semiconductor materials for application in PFC(Zhang et al., 2015a; Zhang et al., 2017 B.; Xia L. et al., 2016; Zeng et al., 2018b; Xie et al., 2018; Lu et al., 2019). A hybrid EF-PFC system was recently reported to introduce an oxygen vacancies-rich rGO/BiOI (rGO/BiO1−xI) photoanode, which possess the advantages of excellent light-harvesting ability, superior charge carriers separation and transfer performance (Hu et al., 2020). Similar advantages are achieved by Z-scheme heterostructured photoanode Ag3PO4@g-C3N4, which ensured an effective separation of charge carriers to achieve a good PFC performance and also maintained a high redox ability to contribute the degradation of organics (Yu et al., 2020). Another novel Z-scheme photoanode WO3/ZnO/Zn has also been recently reported for photodegradation of sunset yellow and phenol (Lam et al., 2020).
Graphitic carbon nitride is a promising material with its visible light activity, tunable bandgap and low cost. The severe charge recombination in the materials limits its wide applicability in the photocatalytic regime. Numerous methods have been reported to reduce its charge recombination and thus enhance its efficiency. Boron doped g-C3N4 synthesized via addition of H3BO3 in urea varied the nanostructure and enhanced its activity towards photocatalytic hydrogen generation. Another report revealed synthesis of g-C3N4 by addition of cyano groups on its surface, which enhances the charge separation and transport in g-C3N4, which again enhanced its performance in hydrogen evolution reaction (Wang X. et al., 2021; 2022a; 2022c). Another visible active materials BiVO4 has been widely studied owing to its suitable band edge positions, stability and low cost. But the use of BiVO4 is limited due to its short hole diffusion length and poor electron transport properties. Many works have been reported to overcome this drawback and make use of BiVO4 as a photoanode in PEC and PFC systems. Songcan Wang and his group recently reported a MoO3-x/BiVO4 photoanode, where the oxygen vacancies in MoO3-x improved the electron mobility in the heterostructure (Wang S. et al., 2020; Wang et al., 2021 S.; 2022b). A novel hybrid photoanode recently reported by a few groups incorporates a PVC with conventional photoanode. Zeng et al. introduced a WO3/BiVO4—Si PVC photoanode and have designed a self-sustaining monolithic photoelectrocatalytic/photovoltaic (SMPP) system, which efficiently degraded tetracycline hydrochloride with a commendable current and hydrogen evolution (Zeng et al., 2018b). TOC and TN were removed by Zhang et al., in 2018 by employing a WO3-Si PVC photoanode (Zhang Y. et al., 2018). Recently Li et al. have come up with a BiVO4/Fe2O3-Si PVC photoanode in PFC, using MB as a model pollutant. With an additional bias voltage, the generation of superoxide radical could be enhanced by 9 times. The maximum power density of the system achieved an appreciable leap owing to the enhanced ROS generation (Li L. et al., 2019). The hybrid photoanodes enable wider bandgap absorption and additional bias voltage for enhanced generation of ROS along with an improved PFC efficiency.
3.2 Materials for cathode
As compared to anode materials, there has been a dearth of research on cathode materials and their influence on PFC. Single photoelectrode systems (SCSP and DCSP) occupy more space in the timeline of PFC research and hence the Pt-based cathode materials were commonly employed. Due to the major drawback of Pt around the concern of cost and low radical generation efficiency, many photocathode materials like Buckypaper, Cu2O, ZnO, ZnIn2S4, WO3, FeVO4, and CoFe2O4, etc. Were also explored by the researchers (Chen et al., 2012; Sfaelou et al., 2012; Bai et al., 2016; Liu et al., 2016; Nahyoon et al., 2019a; Rabé et al., 2019a; 2019b; Xu et al., 2019a; Zhang Y. et al., 2019; Wang et al., 2019; Xu and Xu, 2019).
Pt-based cathodes are widely employed in single photoelectrode PFC systems owing to their high activity towards oxygen reduction reaction (ORR). Pt was used in combination with carbon/TiO2 as a cathode supported on carbon cloth. (Antoniadou and Lianos, 2009; Sfaelou et al., 2015; Xie and Ouyang, 2017; Xie et al., 2018; Jiao et al., 2019). Pt-decorated Si PVC, Pt modified Buried Junction Silicon (BJS), have also been utilized as cathode, where a Si PVC has been incorporated with Pt cathode for efficient charge generation and transfer (Chen et al., 2014; Xia L. et al., 2016; Zhao et al., 2017; Zeng et al., 2018b). The search for a low-cost alternative for Pt led the researchers towards copper based, carbon-based and graphene-based materials which provide similar electrical conductivity and stability as Pt (Canterino et al., 2009; Sfaelou et al., 2012; Ye et al., 2018; Liu X. H. et al., 2019; Nordin et al., 2019). A recent report analyzed the activity of various cathode materials for PFC. Carbon felt, carbon plate, platinum (Pt)-loaded carbon paper, stainless steel mesh and activated carbon flakes were studied. Due to the high surface area and porous nature, activated carbon flakes showed improved efficiency towards RR120 dye degradation and power generation (Mariaswamy et al., 2020). The reports on PFC, which employs cathode materials other than Pt, have been presented in Table 3 for quick view. Photocathode active PFC utilizing BiOCl as photocathode and Pt as anode with Furfur incorporated as fuel has recently been reported (Wang et al., 2020d). Later the scope of having a p-type photocathode that can act complementary with the n-type photoanode and simultaneously photogenerated charge carriers was widely accepted and studied. The p-type semiconductors with a low bandgap enable the absorption of a wider spectrum extending towards the visible region. Photocathode employed PFCs also have the advantage of better ROS generation and multiple pollutant degradation. Nevertheless, the low stability and constraints of having a more negative Fermi level than photoanode limit the choice of cathode materials. A heterojunction photocathode of n-type Fe2O3 and p-type NiO has been successfully employed in a recently reported PMFC (Zhang D. et al., 2020) (Figure 7). Oxides and sulfides are the most studied photocathode materials due to their cost effectiveness and ease of synthesis. Cu2O is the first reported photocathode material that efficiently absorbed visible light along with WO3 photoanode. The credit of enhanced performance of the cell was attributed to mismatched Fermi level positions of WO3 and Cu2O (Chen et al., 2012). On similar lines, Cu-based oxide materials and their nanostructures have been of great interest to the scientific society due to their Fermi level position, visible light photoactivity and low cost. Cu-based photocathode has been employed in PFC for hydrogen production along with electricity generation and pollutant degradation by Wu et al. (Li J. et al., 2013; Wu et al., 2015). Cu2O based heterostructures have also been explored to enhance the charge separation and cathodic activity (Li X. et al., 2018; Lu et al., 2019; Wang et al., 2019).
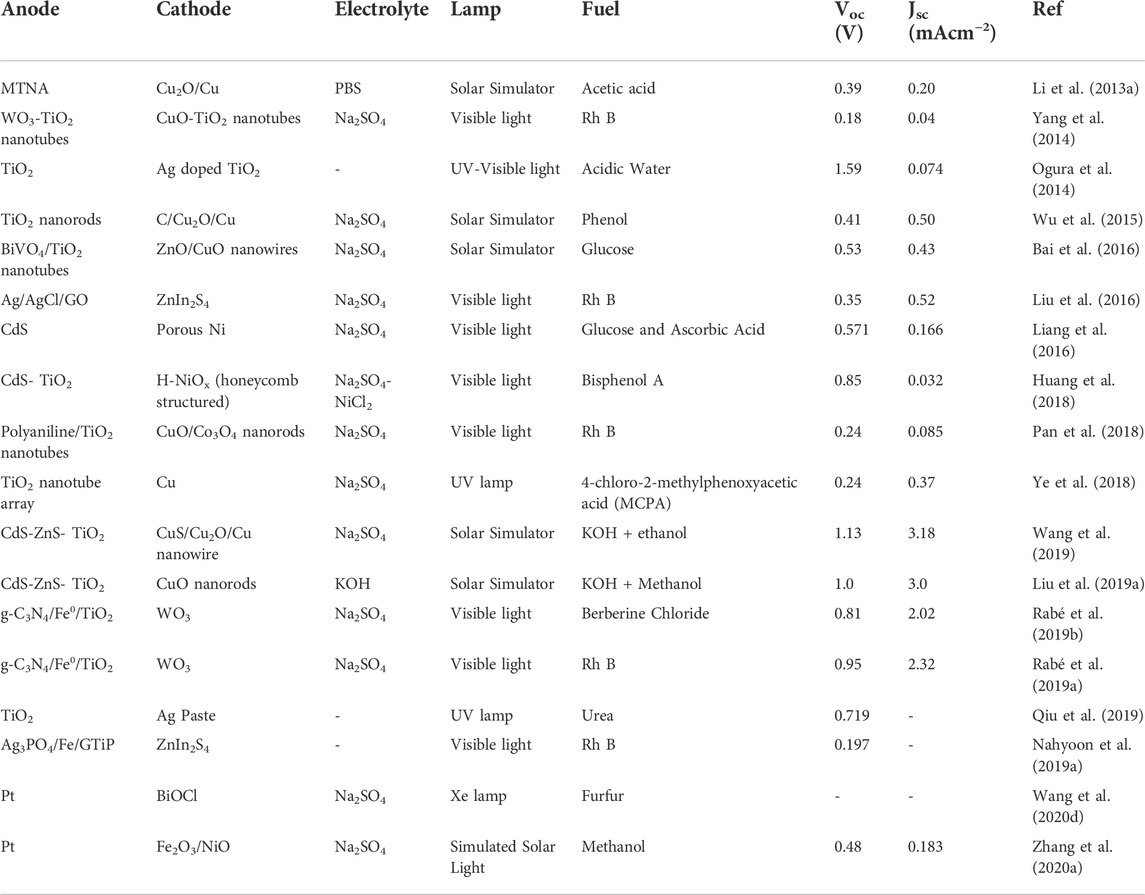
TABLE 3. Quick view on reports of PFC employing cathode materials other than carbon-based materials and Pt.
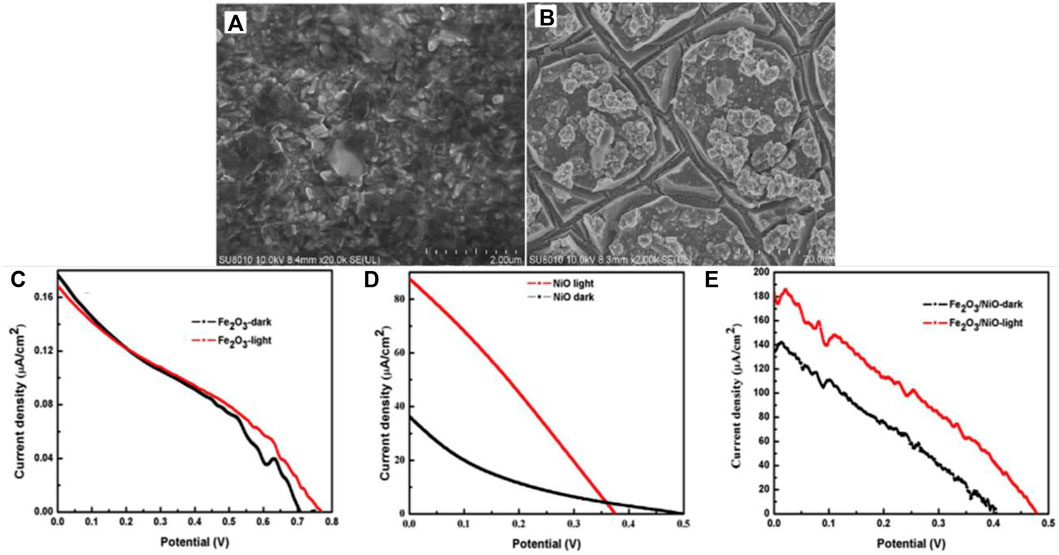
FIGURE 7. SEM images of (A) Fe2O3 (B) Fe2O3/NiO. (C–E) Current density-voltage (I–V) characteristic curves of Fe2O3, NiO and the heterostructure. Reproduced with permission (Zhang D. et al., 2020), Copyright 2020, Elsevier.
4 Developments in applications
The majority of the research on PFCs reports the exploration of various electrode materials and design of PFCs. However, the field of application of PFCs remains less explored. In 2005 when Kaneko et al. coined the term Photo Fuel Cell, it was supposed to produce electric power by simultaneously degrading organic pollutants (fuel) under light irradiation. Along with photocurrent generation, hydrogen evolution was also reported (Kaneko et al., 2005). PFCs were also identified with important applications such as hydrogen peroxide generation, CO2 reduction, heavy metal reduction and sensor applications, other than electricity generation and wastewater treatment (Figure 8) (Wu et al., 2015; He et al., 2018; Andrade et al., 2019; Du et al., 2019; Jiao et al., 2019; Xiao et al., 2019).
4.1 Hydrogen evolution
Generation of electricity along with wastewater treatment can be considered as the primary application of a PFC system. Kaneko et al. and Antoniadou et al. reported the hydrogen evolution from PFC design (Kaneko et al., 2005; Antoniadou et al., 2008). These reports are based on early H2 evolution as a result of photoelectrochemical reactions in PFCs. Since then, the major variation in the design of PFCs, capable of producing hydrogen, is the sealed cathode compartment. A process known as “photoelectrochemical reformation” has been used to describe the breakdown of organic materials. In this process, a photocatalyst anode absorbs sunlight to produce electron-hole pairs, which are then used to photoelectrochemically oxidize organic materials by generating holes as shown in Eq. 17 (Patsoura et al., 2007; Chiarello et al., 2009). Through an external circuit, generated electrons are transferred to the cathode where they eventually react with H+ to make hydrogen as in Eq. 18.
The overall reaction:
The photoelectrochemical reformation may produce usable energy forms like hydrogen and electricity while also cleaning up waste organic material (Strataki and Lianos, 2008; Esposito et al., 2012). Hydrogen evolution was reported in PFC design by providing an electrical biasing (Seger et al., 2012; Liang et al., 2016; Sfaelou et al., 2016). Hydrogen was not catalytically produced in these reports. In other words, electrical biasing was introduced between the electrodes to carry out PEC hydrogen generation. The first report on the simultaneous generation of hydrogen and electricity by utilizing organic pollutants as fuel was by Tang et al., in 2014. In the proposed RDPFC design, TiO2 was employed as the photoanode and Pt as the cathode. The bias required for the reaction kinetics was sequestered from chemical bias produced by the difference in charge of electrolytes, NaOH and H2SO4, anolyte and catholyte, respectively. The group also proposed a Nickel foam-based cathode capable of replacing Pt cathode for catalytic hydrogen generation. Various Ni based substrates has been reported and evaluated for the H2 generation and the Ni foam. (Figure 9) (Tang et al., 2014, 2016).
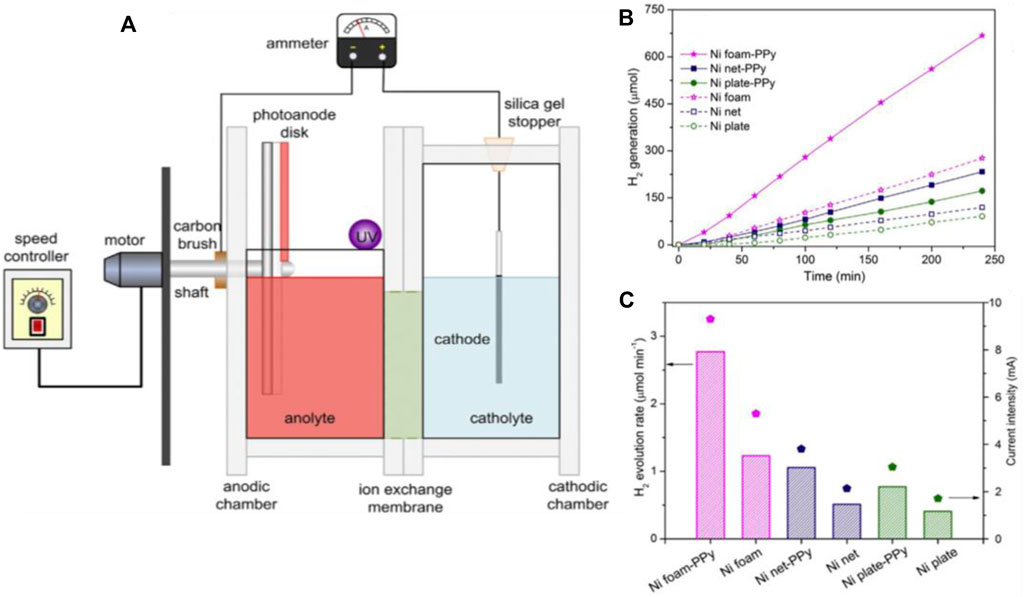
FIGURE 9. (A)Photocatalytic reactor with rotating disc electrode for simultaneous generation of hydrogen and electricity. (B) The catalytic evolution of H2 by untreated or PPy modified three Nickel substrate structures. (C) The H2 generation rate and the current flow with several cathodes Reproduced with permission (Tang et al., 2016), Copyright 2016, Elsevier.
In 2015, we came across the first and only report on a dual photoelectrode PFC that generates hydrogen. It is already known now that in PFC, illumination of semiconductor results in the generation of electrons and holes followed by their separation giving rise to a photovoltage. For n-type semiconductor under light illumination, photo-generated holes are the majority for oxidation. In contrast, for p-type semiconductor under light illumination, photo-generated electrons are the majority for reduction. This photovoltage difference can be exploited for the oxidation of pollutants at photoanode and hydrogen production at photocathode. n-type TiO2/CdS/CdSe photoanodes and p-type Cu2O were employed to generate sufficient bias based on their difference in Fermi energy levels (Wu et al., 2015). A hybrid system with a combination of PFC-PEC systems has been employed successfully for hydrogen generation by Liu et al. Utilization of TiO2 as photoanodes for both cells and Pt on carbon cloth as air-breathing cathode in PFC and Pt as the cathode in PEC (Liu et al., 2017) lead to important findings. However, all the solar light active systems suffer from the challenge of its applicability throughout the day. The complete day operation of PFC can be achieved by the hybrid system design incorporating a mechanism to hold the charge generated during light and then release it in dark. A green supercapacitor assisted photocatalytic fuel cell system with a chemical bias, which aimed for storing photoelectrons by supercapacitors (2 mg cm−2) under light irradiation and discharging for hydrogen production under dark to enable continuous hydrogen production has been recently reported. Even though the objective of the whole system was hydrogen evolution even in dark conditions, the team initially failed to achieve it. The group investigated the possible reasons for the failure and arrived on the significance of cathode potential. Cathode potential (-0.25 V vs. Ag/AgCl) was much higher than the onset hydrogen evolution potential (-0.35 V vs. Ag/AgCl) in dark. The system was modified accordingly and a sustainable hydrogen production under illumination (32 μmol L−1) and in dark (13 μmol L−1), with TiO2 photoanode, Ni-PPy cathode was achieved. The report not only emphasis on the sustainable hydrogen generation of a hybrid system, but also conveys the importance of electrode potentials for the hydrogen evolution reactions. (Zhang et al., 2021). The photogenerated electrons in the photoanode are carried to the cathode by the internal bias created by the difference in Fermi levels between the electrodes in a standard PFC. Unfortunately, the bias potential generated by the Fermi level difference is restricted by the photoelectrode’s conduction band position, which is always too small. A hybrid system which can tackle these challenges and efficiently produce hydrogen has been recently reported. The novel HTPFC composed of a front STNR, a rear silicon solar cell (SSC), and a Pt cathode. The STNR and SSC were built as a monolithic photoanode, with the STNR being able to create electron-hole pairs when activated by high energy photons (>2.94 eV). The SSC may be excited by transmission light to provide a bias potential, which will facilitate electron transport from the STNR to the Pt cathode, resulting in hydrogen generation. The system was capable of achieving degradation of TC with a removal ratio of 94.3% after operation for 1.5 h and an average hydrogen generation rate of 28.8 μmolh−1cm−2. The HTPFC’s higher performance is attributed to its fascinating electrical features, the STNR’s stability, and the rear SSC’s enhanced light exploitation and internal bias (Zeng et al., 2020).
4.2 CO2 reduction
The ever-increasing energy demand and environmental impact of increasing CO2 emission stress the need for a renewable energy source. CO2 reduction mechanisms have been considered as a necessity by researchers around the globe. In this regard, efficient CO2 reduction mechanisms based on various PFC designs can offer a great deal with simultaneous electricity generation.
In 2014, Morikawa et al. reported a reverse photo fuel cell that employed WO3 and layered double halides (LDH) based on Zn, Cu and Ga as photoanode and photocathodes, respectively. The designed system was able to convert CO2 to methanol and oxygen, which are the fuels for a conventional fuel cell. The production of fuels as byproducts gained the system the name ‘reverse’ PFC (Morikawa et al., 2014). Recently, PFC designs incorporated with a CO2 photoreduction reactor have been reported by Long Jiao et al. and Fengjia Xe et al. TiO2 photoanode and Pt cathode have been employed in the PFC. At the same time, TiO2 is used in the CO2 photoreduction reactor. The integrated design photo reduces CO2 as an initial step and produces methanol as a byproduct which acts as fuel for the downstream PFC. In the second step, the methanol is degraded in PFC and electric power is produced. So, on the whole, CO2 is photo reduced to produce direct electric power, which addresses the issue of energy demand as well as CO2 reduction. In this design, even the unreacted CO2 from the upstream photoreduction reactor can be converted to hydrocarbon fuels at photoanode of downstream PFC (Jiao et al., 2019; Xie et al., 2019). An improved photocatalytic reduction of CO2 to CH4 in a thin-film RDPFC reactor have been recently reported. The yield of CH4 in this system was measured to be 7.62 μmol g−1 h−1, which was achieved by the diffusion of CO2 through the thin film and the high solubility of CO2 in the aqueous electrolyte solutions. The thin film RDPFC system was capable of a power output of 10.5 μW cm−2. The improved efficacy of the system is attributed towards the thin film RDPFC design which incorporated a TiO2– NT rotating disc photoanode (Zhang et al., 2020c).
A CO2 self-driving and self-recycling photocatalytic fuel cell (PFC) system was recently reported by Zhang et al. Comparing the carbon neutral PFC system to the traditional photocatalytic system, the PFC system increased organics removal by 40% and added 6.7 μmolg−1h−1 additional C1 fuel yield rates. The CO2 generated at anode upon wastewater degradation was continuously supplied to rotating cathode (Figure 10). The CO2 reduction reaction occurring at the cathode accelerated the electron−hole separation in photoanode and facilitated the formation of C1 fuel in the cathode (Zhang et al., 2020b). A recent report which investigated the role of CO2 in the photocatalytic fuel cell drew many interesting scientific conclusions. The PFC system with TiO2 as the photoanode and methanol as a fuel under acid condition was tested by making the anode electrolyte saturated with CO2. It was revealed that the performance of the photocatalytic fuel cell can be improved at low fuel concentration by the presence of CO2. When there was no CO2 in the anode electrolyte, the OCV was 0.97 V, the SCC was 0.107 mA cm−2, the MPD was 0.052 mW cm−2. But, when the anode electrolyte was saturated with CO2, although the OCV was close to the case without CO2, the SCC and MPD were 0.128 mA cm−2 and 0.072 mW cm−2, respectively, both of which were higher than the case without CO2. These results deny the conventional wisdom that CO2 as a product should be removed as soon as possible to improve the performance. Youxu et al. also discusses about the plausible reasons towards the interesting phenomena they observed and those reasons are as follows: TiO2 had an adequate conduction band potential of -0.2 V vs. NHE, it was more negative than the thermodynamic potential for CO2 reduction to methanol at pH = 1 (-0.026 V vs. NHE), which will facilitate the photocatalytic reduction of CO2 to organics at the photoanode, which increases the methanol concentration at the catalytic surface to alleviate the mass transfer issue encountered in the low methanol concentration operation, and hinders the recombination of the electron-hole pairs (Yu et al., 2021).
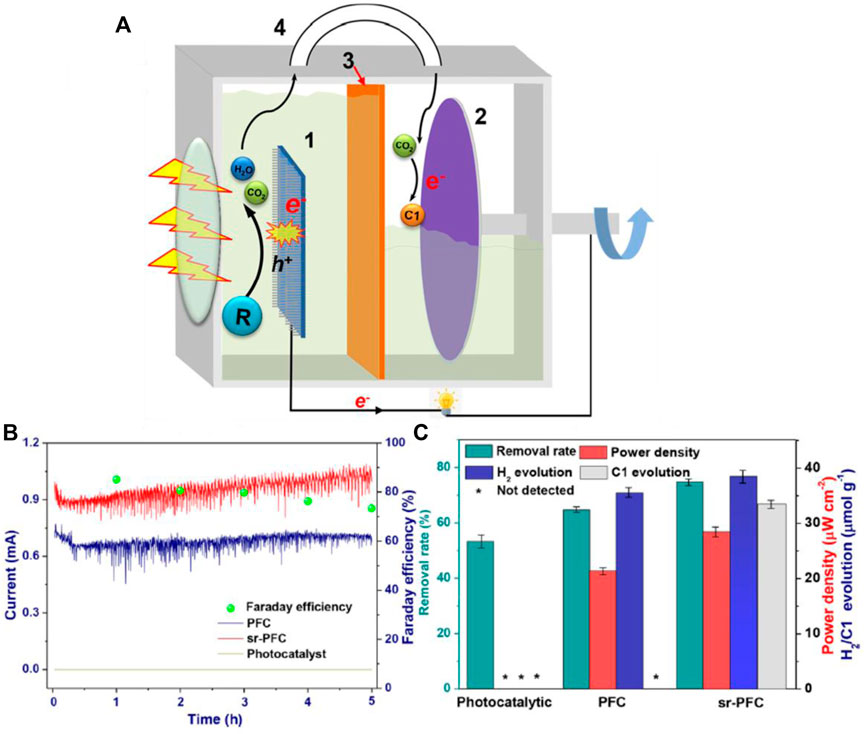
FIGURE 10. (A) Schematic diagram of the experimental setup. 1. TiO2-NT photoanode; 2. Ti3C2 cathode; 3. Cation-exchange membrane; and 4. Gas channel. (B) Current response and Faraday efficiency of CO2 reduction (C) by-product analysis in different operating system within 5 h. Reproduced with permission (Zhang et al., 2020b), Copyright 2020, American Chemical Society.
4.3 Hydrogen peroxide production
Hydrogen peroxide finds its applicability in multiple areas of pharmaceuticals, advanced oxidation processes, Fenton reactions and even as energy storage. It is considered to store chemical energy while being used in hydrogen peroxide fuel cells and even as an oxidant in many other types of fuel cells. Many methods have been adopted for efficient H2O2 production like the anthraquinone oxidation method, electrochemical advanced oxidation technologies and microbial fuel cells. However, the issues related to potential toxicity, high operational costs, the expense of external power supply, complex operation, stringent working conditions and long start-up time due to the presence of bacteria have suppressed the large-scale applications of these technologies.
PFC as a promising technology for efficient H2O2 production has been reported by a few groups recently. Santos Andrade et al. reported two PFC systems with CdS/TiO2 and CdSe/CdS/TiO2 as visible light active photoanodes, respectively and carbon black/carbon cloth as the cathode (Figure 11). NaHCO3 was used as a catholyte which enhanced the water oxidation by HCO3− catalysis and enhanced the H2O2 production in the cathode (Andrade et al., 2019).
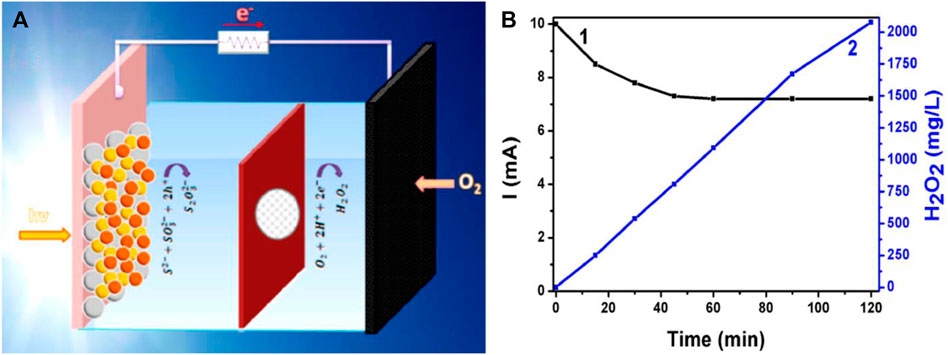
FIGURE 11. (A) Schematic design of the photofuel cell reactor used for H2O2 production. (B)Evolution of the short-circuit current (1) and of the quantity of photoelectrochemically produced H2O2 (2) using a PFC operated with a ternary semiconductor photoanode. Reproduced with permission (Andrade et al., 2019), Copyright 2019, Multidisciplinary Digital Publishing Institute.
A novel mechanism integrating PFC and Fenton reaction assisted by reverse electrodialysis (PREC) has been recently proposed by Peng Xu et al. Reverse electrodialysis (RED) is a novel technology for electricity production. Alternately stacked anion exchange membranes (AEM) and cation exchange membranes (CEM) separated by high concentration (HC) and low concentration (LC) cells are collectively called RED stack. When the stack is supplied with suitable HC and LC solutions, current is generated due to the migration of ions through AEM and CEM. In the PREC reports, ZnO/Zn is used as photoanode and FeVO4/carbon felt (CF) is used as cathode. The photogenerated electrons from the photoanode separate and travel efficiently towards the cathode with an enhanced bias of RED stack and hydrogen peroxide is produced at the cathode by the two-electron reduction of dissolved O2 on the FeVO4/CF surface (Xu et al., 2019b; Xu and Xu, 2019). A PFC system where the in-situ generation of H2O2 is reported, but the generated H2O2 is consumed by the system itself as a source of hydroxyl radical and superoxide radical. The generation of radicals in turn improves the overall efficacy of the system. PFC system was comprised of SSC incorporated Fe2O3/BiVO4 photoanode and graphene felt cathode. The electron-hole pairs are generated upon light irradiation in photoanode and the photogenerated holes oxidize H2O to oxygen and hydroxyl radical owing to its potential. SSC acts as an additional potential supplier in the system. Electrons reaching the graphene felt cathode combines with adsorbed oxygen to produce H2O2. Due to its high hydrophilicity, generated H2O2 is quickly transferred to the solution for radical chain reaction with added Fe ions in the system (Li et al., 2020). A record-high H2O2 concentration of 110 mmol L−1 is achieved by Wenjun Fan et al., where the group have employed polyterthiophene (pTTh), a metal free narrow-bandgap polymeric semiconductor, as cathode is an efficient photocathode for H2O2 production in alkaline solution (Fan et al., 2020). A work has studied the photoelectrochemical production of hydrogen peroxide and the direct use of the produced material for the degradation (decolorization) of three common dyes: methylene blue, basic blue 41 and acid orange 7. The reported PFC operated with a CdS-sensitized mesoporous titania photoanode and a simple carbon cloth cathode carrying a hydrophobic layer of mesoporous carbon (carbon black). Hydrogen peroxide was produced in the cathode compartment of the PFC by atmospheric oxygen reduction, with a very high Faradic efficiency nearing 100%. The system was highly effective since hydrogen peroxide production rate was close to the theoretically expected value. The maximum quantity of H2O2 produced in the present case after 4 h of continuous operation was 1,200 mg L−1 which corresponds to about 35 mmol L−1. These results open a route for the conversion and storage of solar radiation in the form of chemical energy (Dhawle et al., 2021).
4.4 Heavy metal reduction
Wastewater treatment has been a hot topic for many years for the scientific world due to its crucial role in environmental reconditioning. We have numerous reports based on biological treatment, membrane technology, chemical and electrochemical techniques that can effectively remove pollutants. However, these techniques completely ignore the ways of energy recovery from wastewater. The PFC designs find their importance in the area where energy recovery and pollutant degradation are steered simultaneously. The presence of heavy metals in water has been realized as a huge problem, related to the fact that removal of heavy metals is a tedious task. Most of the heavy metal reduction techniques ignore the chemical energy which can be recovered from the same. Recently Xiao et al. reported a PFC which can simultaneously degrade Bisphenol A and reduce Cr (VI) along with electricity generation. The report is considered to be the first report on using dopamine modified carbon as cathode and BiVO4 as photoanode for heavy metal reduction using PFC. As BPA gets photocatalytically degraded in anode chamber by BiVO4, Cr (VI) gets reduced to less mobile and less toxic Cr (III) in the cathode chamber. The schematic illustration of the PFC working is as shown in Figure 12. Even though a PFC design is used, the reports do not state that the heavy metal reduction is purely based on the photocatalytic activity. Adsorption property and surface properties of cathode plays a vital role in reduction mechanism (Xiao et al., 2019). A complete multifunctional PFC which can degrade pollutants, reduce heavy metal and generate power was introduced later by Liu et al. The device degraded model pollutant MB and reduced Cr (VI) with an enhanced power generation.
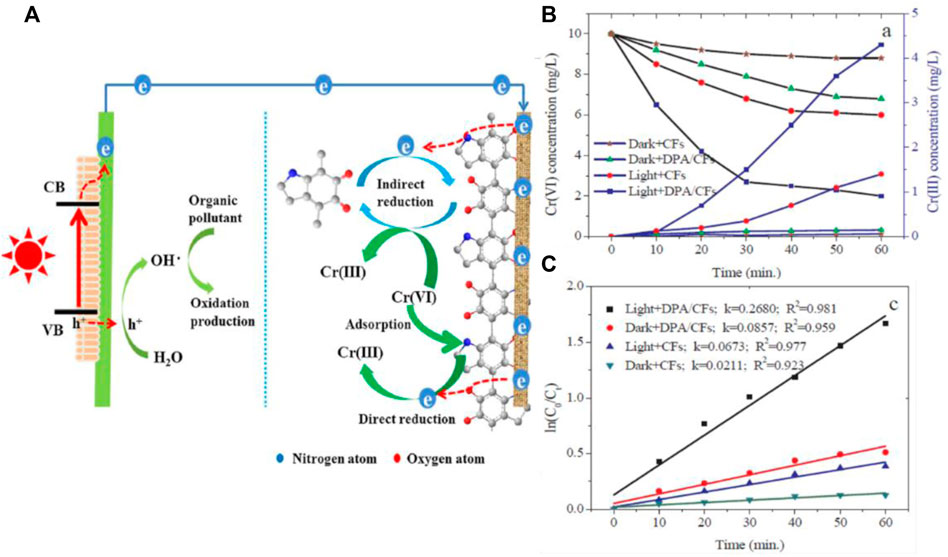
FIGURE 12. (A) Schematic illustration depicting the simultaneous degradation of Bisphenol A and Cr (VI) reduction. (B) The concentrations of Cr (VI) and Cr (III) in the cathode chamber. (C) The Cr(VI) reduction kinetics with the carbon felt (CF) and carbon felt cathode modified by dopamine (DPA/CF). Reproduced with permission (Xiao et al., 2019)Copyright 2019, Elsevier.
TiO2 and graphite were employed as photoanode and cathode, respectively, in separate chambers. Complete degradation of MB and 96.8% removal of Cr (VI) with a cathodic efficiency of 95.1% was achieved, with a maximum power output being 1 W m−2. Cr(VI) reduction is thermodynamically more advantageous than that of oxygen, and it could draw more photogenerated electrons to the cathode to thus render a higher electric power, better degradation and enhanced removal percentage (Liu X. H. et al., 2019). A synergetic photocatalytic degradation of organic dye (X3B) in anode and the reduction of heavy metal Cr(VI) in cathode are carried out in an improved dual-chamber PFC device with TiO2 and core–shell structured Ag@Fe2O3 nanoparticles as photoanode and cathode materials in a recent report. Ag@Fe2O3 shows the highest catalytic activity to the reduction of Cr (VI) with an apparent kinetic constant of 0.058 min−1, even much better than commercial Pt foil. It is worth noting that the kobs value of Fe2O3 is only lower than that of Ag@Fe2O3 but much higher than those two noble metals (Pt and Ag), indicating that Fe2O3 truly has a considerable catalytic ability for the reduction of Cr (VI). The improved performance in cathodic reduction of Cr(VI) with Ag@Fe2O3 cathode can be attributed to directional transport channels supplied by Ag cores and desirable catalytic activities of Fe2O3 shells (Sun et al., 2022).
4.5 Sensor applications
Photocatalytic fuel cell-based sensors are a very recent advancement in the field of applications of PFC. The first report on PFC based sensor was published in 2016 by Kai Yan et al. The reported self-powered sensor consisted of Ni (OH)2/CdS/TiO2 photoanode and hemin graphene cathode. The group successfully developed a glucose sensor with a detection limit as low as 5.3 µM. The H2O2 reduction at the cathode and the visible light activity made the sensor a significant milestone in the application pathway of PFC (Yan et al., 2016).
One of the major drawbacks in combining the practicality of photocatalysis in sensor platforms is the non-selective nature of the process. Hence the same group came up with a PFC sensor, which incorporated a molecularly imprinted polymer (MIP) for the selective sensing of p-Nitrophenol. Graphene modified GCE was employed as the anode, which oxidised the Ascorbic acid and PbS quantum dot modified GCE was used as the photocathode, which reduced the p-NP under irradiation. The PFC-based MIP sensor achieved a p-NP detection limit of 0.031 µM (Yan et al., 2017). MIP integrated PFC based novel self-powered biosensor was constructed to achieve sensitive and specific detection of aflatoxin B1 (AFB1). MoS2–Ti3C2TxMXene (MoS2–MX) served as the photoanode material for the first time by forming a heterojunction structure, which can enhance the photocurrent by about 3-fold and greatly improve the photoelectric conversion efficiency. The self-powered biosensor showed a wide dynamic range of 0.01–1,000 ng ml−1 with a detection limit of 0.73 pg ml−1 with MIP and PFC combined to act as recognition and signal conversion elements respectively (Jin et al., 2021). A work which utilises the material property of Ni-based materials to form Ni2+/Ni3+redox couple for glucose detection. Dual system based on PEC and PFC techniques was studied by utilising Ni(OH)2/TiO2 as anode and Pt cathode, with a detection limit of glucose as low as 5 µM (Yang et al., 2017). Later in 2018 aptamer-based PFC sensing application was reported by Kai Yan et al. A visible, membrane less, H2O2 PFC system was developed by the group for sensing PCB77, which potentially causes cancer and many adverse health issues. Au nanoparticles decorated g-C3N4 nanosheet and hemin graphene nanocomposites were used as photoanode and cathode, respectively. Binding aptamer (5′-SH-(CH2)6-GGC GGG GCT) immobilised on photoanode couples with PCB77 present in the solution and affects the electron transfer, which in turn enhances the sensitivity and selectivity of the system. The same group came up with MIP based sensor for Bisphenol-A sensing with MIP/g-C3N4 on FTO as anode and Pt cathode. The binding property of MIP and efficient photocatalytic activity of carbon nitride synergistically brought down the detection limit of BPA to 1.3 µM (Yan et al., 2018b; 2018a). Few reports on PFC sensors for enhanced detection of pharmaceuticals and enzymes (affecting both humans and animals) were reported in 2019. The first report on a dual photoelectrochemical-PFC sensor for sensing of microcystin-LR, a potential toxin produced by cyanobacteria present in drinking water, was proposed by Du et al. This system had a detection limit of 0.67p.m. and employed TiO2 nanoparticles and nitrogen-doped graphene with BiOBr as anode and cathode respectively (Du et al., 2019). Kanamycin, an antibiotic that causes side effects related to hearing and balance problems and can even cause kidney-related health issues, was efficiently detected using an aptamer-based PFC sensor, which employed graphene doped BiVO4 as photoanode and Pt as the cathode. Aptamer-based sensing helped in selectivity and a detection limit of 1 nM was achieved (Ji et al., 2019). A similar antibiotic, Bleomycin, which possesses severe concomitant effects related to lungs, was detected using a visible light activated PFC sensor by Mengjun et al. Aptamer modified CdS-In2S3 and Pt was utilised as photoanode and cathode for the efficient sensing with a detection limit of 1 nM(Sun et al., 2019).
Recently, a Tyrosinase PFC sensor which finds its application in melanoma diagnosis was developed by Kai Yan et al. with a detection limit as low as 0.005 U mL−1 g-C3N4/Bi2S3 and hemin graphene was used as photoanode and cathode, respectively (Yan et al., 2019). A photoelectrochemical (PEC) oxidation of glucose at tungsten trioxide nanoplate (WO3 NP) electrode for glucose sensing application and photo fuel cell (PFC) application has been reported recently. The WO3-based PEC sensor shows high sensitivity of 68.15 μA cm−2 mM−1 in glucose detection for the concentrations in the range of 0.1∼0.5 mM. Moreover, electricity can be extracted from photo-oxidizing of glucose on WO3 NP electrode in a PFC device and the output strongly depends on the concentration of glucose fuel (Zhang B. et al., 2019). A Fe2O3 nanorod-based PFC sensor, which shows excellent performance in glucose detection for the concentrations in the range of 0.5–2.5 mM with a sensitivity of 3.53 μAcm−2mM−1. The report not only realizes the electricity generation from renewable solar energy and bioenergy but also accomplishes the detection of glucose by self-power (He et al., 2020). The self-powered PFC sensors reported so far faces the challenge of varying intensity of light source, which will affect the sensing parameters and the outputs. A ratiometric PFC design, which is capable of addressing the flux variations on incident light was reported by Xiaoling Yao et al. (Figure 13). A highly selective detection of 17β-estradiol (E2) is realised, based on a dual-channel PFC constructed with two photoanodes which could effectively avoid the fluctuation of the light intensity. E2 was quantified via the ratio of output power density values from dual photoanodes. The sensing signal was linearly related to the logarithm of E2 concentration in the range of 1–500 nM, with a detection limit (3S/N) of 0.12 nM (Yao et al., 2020). The development of PFC self-powered sensors from model analyte sensing to various pollutants detection in a short period of time projects its potential to become the simplest and self-powered replacement for conventional sensors.
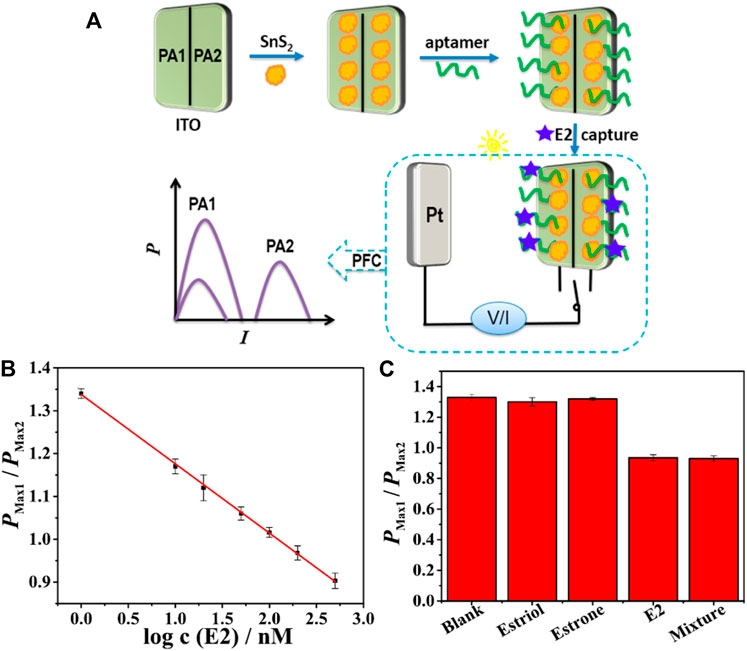
FIGURE 13. (A) Schematic illustration of fabrication process of dual-channel PFC for ratiometric self-powered aptasensing(B) Calibration curve for E2 detection by the proposed ratiometric self-powered aptasensor based on dual photoanodes. (C)Histogram for Pmax1/Pmax2 values of the proposed sensor toward 200 nM estriol, estrone, E2, and their mixture. Reproduced with permission (Yao et al., 2020), Copyright 2020, American Chemical Society.
5 Conclusion and future perspectives
A chronological growth of Photocatalytic Fuel Cells on the grounds of system design, materials used and potential applications has been consolidated in the article. The design of the PFC system has been discussed as SCSP design, DCSP design, SCDP design, Triple chamber system, MFC design, RDPFC system and Hybrid systems based on the number of chambers, characteristics of the electrodes and interaction of the overall processes. Single Chamber Single Photoelectrode system was the design of the first ever reported PFC system. It still stands out as the most explored design accounting to its simplicity in design and efficient charge transfer properties. In the case of SCSP, the simplicity in design also points towards the narrowing of applicability in various areas. The upcoming designs of dual chamber PFC systems shed light towards a bright horizon of multiple applications on a single system such as simultaneous degradation of various dyes in the anodic and cathodic chamber, degradation and heavy metal reduction simultaneously in each chamber, degradation in one and hydrogen evolution in the other. Triple chamber systems which work on SGP, RDPFCs, which incorporate photocatalysis along with properties of thin film surfaces and MFCs that rely on microscale optofluidics are promising system designs that enjoy great room for further exploration of scientific society. Hybrid systems can be stated as the future of PFCs, where various well-explored systems and PFC are combined. Fenton-PFC systems, SMPP systems, PFC-PEC systems, PFC-PVC systems, CO2 reduction-PFC systems, Micro supercapacitor-PFC systems and RED-PFC systems have been employed for various applications ranging from degradation along with electricity generation to H2O2 production, TOC and total nitrogen removal and even on the grounds of energy storage. Further, the reports on novel designs of PFC primarily emphasize its novelty and enhancing the efficiency of the PFC system. In our view, the novel designs from research groups should pay attention to exploiting the arena of various applications rather than merely focusing on enhancing primary PFC mechanisms. Even though design plays a vital role in the efficiency of the PFC system, a proper selection of materials also contributes equally towards the overall performance of the system.
Anode and cathode material properties play a prominent role in the efficiency of the system. TiO2 can still be considered as the champion material as photoanode and Pt has been widely employed as the cathode. Nanostructures of TiO2 and narrow bandgap material-TiO2 heterostructures have proven to be great anode candidates with their efficient electron transfer and wider absorption spectrum. ZnO, BiVO4, WO3, Fe2O3 have also shown better charge separation and transfer. Meanwhile, cathode materials lack selection choices due to the need for better electronic conductivity, stability and corrosion resistance in photoelectrode systems and suitable band edge positions as that of the anode in dual photoelectrode systems. Carbon-based materials were found to possess properties that could allow them to be considered an optimum replacement for costly Pt electrodes. As dual photoelectrodes system came into the scene, the cathode research also acquired a velocity and many p-type semiconductor materials such as sulfides, Cu-based materials, spinel-ferrite structures have been studied so far. Even though there are numerous reports on various materials other than TiO2 and Pt, the research on materials implemented for electrodes still has to be considered insufficient when compared with the booming material research in the photocatalysis regime. The scope of exploring materials and their unique properties for PFC research is a great arena for researchers around the globe.
PFC was initially employed as a system for simultaneous pollutant degradation and an electric power source. As the timeline progressed, the arena of application widened and has since been extended towards H2 and H2O2 generation, CO2 and heavy metal reduction and sensor applications. The environmental importance of PFC is understood when we realize that all these applications exist along with pollutant degradation and electricity generation. Even though the concept has immense potential in various applications, the scientific community should emphasize on the utilization of natural solar irradiation so that a much sustainable technology can be established. From the above discussion the key take home points can be consolidated as 1) Hybrid PFCs are the future of PFCs and the scientific community should be focusing more on the synergy of PFCs along with other potential systems such as Fenton systems, photovoltaic systems etc. 2) The photoelectrode material research should be more concentrated on low cost, highly efficient materials and a proper focus has to be placed on the visible light active photoelectrodes and p-type photocathodes 3) The energy consuming light source such as UV-light, Visible lamp, solar simulator etc. should be used as a preliminary approach towards PFCs and the ultimate objective should be a system which is active under natural solar irradiation, which will substantiate the fundamental idea of PFC research. Proper selection of design and materials play a vital role in applications and focusing on those that can deliver high impact works on the applications of PFC.
Author contributions
SJ wrote the article. BG and SS contributed towards original idea, discussion, analysis and edited the article. WN helped in discussion, editing and proof reading the article.
Acknowledgments
BG thanks for funding from the European Union’s Horizon 2020 research and innovation programme under the Marie Skłodowska-Curie grant agreement No. 847413 for funding. Scientific work published as part of an international co-financed project founded from the programme of the Minister of Science and Higher Education entitled “PMW” in the years 2020–2024; agreement no. 5005/H2020-MSCA-COFUND/2019/2. SS would like to acknowledge University Grants Commission (UGC), DST Solar Energy Harnessing Centre - DST/TMD/SERI/HUB/1(C), DST/TMD -EWO/WTI/2K19/EWFH/2019/122, SERB grant (SPG/2021/002867) and SERB project (EMR/2017/000794). WN is grateful to National Science Centre (Poland, NCN) for fundingUMO-2016/23/B/ST4/02868. SJ would like to acknowledge Anna Centenary Research Fellowship (CFR/ACRF/19157291139/AR1) for providing the financial support to carry out the research work.
Conflict of interest
The authors declare that the research was conducted in the absence of any commercial or financial relationships that could be construed as a potential conflict of interest.
Publisher’s note
All claims expressed in this article are solely those of the authors and do not necessarily represent those of their affiliated organizations, or those of the publisher, the editors and the reviewers. Any product that may be evaluated in this article, or claim that may be made by its manufacturer, is not guaranteed or endorsed by the publisher.
References
Ammar, S. H., Shafi, R. F., and Ali, A. D. (2020). A novel airlift photocatalytic fuel cell (APFC) with immobilized CdS coated zerovalent iron (Fe@CdS) and g-C3N4 photocatalysts film as photoanode for power generation and organics degradation. Colloids Surfaces A Physicochem. Eng. Aspects 602, 125164. doi:10.1016/j.colsurfa.2020.125164
Andrade, T. S., Papagiannis, I., Dracopoulos, V., Pereira, M. C., and Lianos, P. (2019). Visible-light activated titania and its application to photoelectrocatalytic hydrogen peroxide production. Mater. (Basel) 12, 1–13. doi:10.3390/ma1224238
Antolini, E. (2019). Photoelectrocatalytic fuel cells and photoelectrode microbial fuel cells for wastewater treatment and power generation. J. Environ. Chem. Eng. 7, 103241. doi:10.1016/j.jece.2019.103241
Antoniadou, M., Bouras, P., Strataki, N., and Lianos, P. (2008). Hydrogen and electricity generation by photoelectrochemical decomposition of ethanol over nanocrystalline titania. Int. J. Hydrogen Energy 33, 5045–5051. doi:10.1016/j.ijhydene.2008.07.005
Antoniadou, M., Kondarides, D., Labou, D., Neophytides, S., and Lianos, P. (2010). An efficient photoelectrochemical cell functioning in the presence of organic wastes. Sol. Energy Mat. Sol. Cells 94, 592–597. doi:10.1016/j.solmat.2009.12.004
Antoniadou, M., and Lianos, P. (2009). Near Ultraviolet and Visible light photoelectrochemical degradation of organic substances producing electricity and hydrogen. J. Photochem. Photobiol. A Chem. 204, 69–74. doi:10.1016/j.jphotochem.2009.02.001
Ayekoe, C. Y. P., Robert, D., and Lanciné, D. G. (2017). Combination of coagulation-flocculation and heterogeneous photocatalysis for improving the removal of humic substances in real treated water from Agbô River (Ivory-Coast). Catal. Today 281, 2–13. doi:10.1016/j.cattod.2016.09.024
Bai, J., Wang, R., Li, Y., Tang, Y., Zeng, Q., Xia, L., et al. (2016). A solar light driven dual photoelectrode photocatalytic fuel cell (PFC) for simultaneous wastewater treatment and electricity generation. J. Hazard. Mater. 311 (5), 51–62. doi:10.1016/j.jhazmat.2016.02.052
Balis, N., Dracopoulos, V., Antoniadou, M., and Lianos, P. (2012). One-step electrodeposition of polypyrrole applied as oxygen reduction electrocatalyst in Photoactivated Fuel Cells. Electrochim. Acta 70, 338–343. doi:10.1016/j.electacta.2012.03.086
Bernabeu, A., Vercher, R. F., Santos-Juanes, L., Simón, P. J., Lardín, C., Martínez, M. A., et al. (2011). Solar photocatalysis as a tertiary treatment to remove emerging pollutants from wastewater treatment plant effluents. Catal. Today 161, 235–240. doi:10.1016/j.cattod.2010.09.025
Canterino, M., Di Somma, I., Marotta, R., Andreozzi, R., and Caprio, V. (2009). Energy recovery in wastewater decontamination: Simultaneous photocatalytic oxidation of an organic substrate and electricity generation. Water Res. 43, 2710–2716. doi:10.1016/j.watres.2009.03.012
Chen, Q., Bai, J., Li, J., Huang, K., Li, X., Zhou, B., et al. (2014). Aerated visible-light responsive photocatalytic fuel cell for wastewater treatment with producing sustainable electricity in neutral solution. Chem. Eng. J. 252, 89–94. doi:10.1016/j.cej.2014.04.046
Chen, Q., Li, J., Li, X., Huang, K., Zhou, B., Cai, W., et al. (2012). Visible-light responsive photocatalytic fuel cell based on WO 3/W photoanode and Cu 2 O/Cu photocathode for simultaneous wastewater treatment and electricity generation. Environ. Sci. Technol. 46, 11451–11458. doi:10.1021/es302651q
Chen, R., Xia, M., Zhu, X., Liao, Q., Ye, D., An, L., et al. (2018). A visible-light responsive micro photocatalytic fuel cell with laterally arranged electrodes. Appl. Therm. Eng. 143, 193–199. doi:10.1016/j.applthermaleng.2018.07.099
Chiarello, G. L., Forni, L., and Selli, E. (2009). Photocatalytic hydrogen production by liquid- and gas-phase reforming of CH3OH over flame-made TiO2 and Au/TiO2. Catal. Today 144, 69–74. doi:10.1016/j.cattod.2009.01.023
Daneshvar, N., Salari, D., and Khataee, A. R. (2004). Photocatalytic degradation of azo dye acid red 14 in water on ZnO as an alternative catalyst to TiO2. J. Photochem. Photobiol. A Chem. 162, 317–322. doi:10.1016/S1010-6030(03)00378-2
Deng, B., Fu, S., Zhang, Y., Wang, Y., Ma, D., and Dong, S. (2018). Simultaneous pollutant degradation and power generation in visible-light responsive photocatalytic fuel cell with an Ag-TiO2 loaded photoanode. Nano-Structures Nano-Objects 15, 167–172. doi:10.1016/j.nanoso.2017.09.011
Dhawle, R., Frontistis, Z., Mantzavinos, D., and Lianos, P. (2021). Production of hydrogen peroxide with a photocatalytic fuel cell and its application to UV/H2O2 degradation of dyes. Chem. Eng. J. Adv. 6, 100109. doi:10.1016/j.ceja.2021.100109
Dong, F., Wang, Z., Li, Y., Ho, W. K., and Lee, S. C. (2014). Immobilization of polymeric g-C3N4 on structured ceramic foam for efficient visible light photocatalytic air purification with real indoor illumination. Environ. Sci. Technol. 48, 10345–10353. doi:10.1021/es502290f
Du, X., Jiang, D., Liu, Q., Hao, N., and Wang, K. (2019). Ingenious dual-photoelectrode internal-driven self-powered sensing platform for the power generation and simultaneous microcystin monitoring based on the membrane/mediator-free photofuel cell. Anal. Chem. 91, 1728–1732. doi:10.1021/acs.analchem.8b05509
Erickson, D., Sinton, D., and Psaltis, D. (2011). Optofluidics for energy applications. Nat. Photonics 5, 583–590. doi:10.1038/nphoton.2011.209
Esposito, D. V., Forest, R. V., Chang, Y., Gaillard, N., McCandless, B. E., Hou, S., et al. (2012). Photoelectrochemical reforming of glucose for hydrogen production using a WO3-based tandem cell device. Energy Environ. Sci. 5, 9091–9099. doi:10.1039/C2EE22560C
Fan, W., Zhang, B., Wang, X., Ma, W., Li, D., Wang, Z., et al. (2020). Efficient hydrogen peroxide synthesis by metal-free polyterthiophene via photoelectrocatalytic dioxygen reduction. Energy Environ. Sci. 13, 238–245. doi:10.1039/C9EE02247C
Fujishima, A., and Honda, K. (1972). Electrochemical photolysis of water at a semiconductor electrode. Nature 238, 37–38. doi:10.1038/238037a0
He, L., Zhang, Q., Gong, C., Liu, H., Hu, F., Zhong, F., et al. (2020). The dual-function of hematite-based photoelectrochemical sensor for solar-to-electricity conversion and self-powered glucose detection. Sensors Actuators B Chem. 310, 127842. doi:10.1016/j.snb.2020.127842
He, X., Chen, M., Chen, R., Zhu, X., Liao, Q., Ye, D., et al. (2018). A solar responsive photocatalytic fuel cell with the membrane electrode assembly design for simultaneous wastewater treatment and electricity generation. J. Hazard Mater 358, 346–354. doi:10.1016/j.jhazmat.2018.07.031
He, Y., Chen, K., Leung, M. K. H., Zhang, Y., Li, L., Li, G., et al. (2022). Photocatalytic fuel cell – a review. Chem. Eng. J. 428, 131074. doi:10.1016/j.cej.2021.131074
He, Y., Zhang, Y., Li, L., Shen, W., and Li, J. (2021). An efficient optofluidic photocatalytic fuel cell with dual-photoelectrode for electricity generation from wastewater treatment. J. Solid State Chem. 293, 121780. doi:10.1016/j.jssc.2020.121780
Hill, J. C., and Choi, K. S. (2012). Effect of electrolytes on the selectivity and stability of n-type WO 3 photoelectrodes for use in solar water oxidation. J. Phys. Chem. C 116, 7612–7620. doi:10.1021/jp209909b
Hu, C., Kelm, D., Schreiner, M., Wollborn, T., Mädler, L., and Teoh, W. Y. (2015). Designing photoelectrodes for photocatalytic fuel cells and elucidating the effects of organic substrates. ChemSusChem 8, 4005–4015. doi:10.1002/cssc.201500793
Hu, L., Liao, Y., Xia, D., Peng, F., Tan, L., Hu, S., et al. (2020). Engineered photocatalytic fuel cell with oxygen vacancies-rich rGO/BiO1−xI as photoanode and biomass-derived N-doped carbon as cathode: Promotion of reactive oxygen species production via Fe2+/Fe3+ redox. Chem. Eng. J. 385, 123824. doi:10.1016/j.cej.2019.123824
Huang, B., Li, N., Lin, W., and Li, H. (2018). A highly ordered honeycomb-like nickel(III/II) oxide-enhanced photocatalytic fuel cell for effective degradation of bisphenol A. J. Hazard. Mat. 360, 578–586. doi:10.1016/j.jhazmat.2018.08.058
Huang, M., Zhou, C., Wen, R., Tian, J., Huang, W., Wei, H., et al. (2022). Membraneless photocatalytic fuel cell with double photoelectrodes for simultaneous electricity generation and pollutant degradation. J. Electrochem. Soc. 169, 026502. doi:10.1149/1945-7111/ac4b21
Ji, W., Yan, K., Chen, Y., Gao, J., and Zhang, J. (2019). A visible light-induced self-powered aptasensing platform for kanamycin detection based on mediator-free photofuel cell. Sensors Actuators B Chem. 292, 129–135. doi:10.1016/j.snb.2019.04.125
Jia, X., Huang, L., Gao, R., Devaraji, P., Chen, W., Li, X., et al. (2020). Improvement of photocatalytic hydrogen generation of leaves-like CdS microcrystals with a surface decorated by dealloyed Pt-Co nanoparticles. Sol. Energy 206, 8–17. doi:10.1016/j.solener.2020.05.073
Jiao, L., Xie, F., Chen, R., Ye, Di., Zhang, B., An, L., et al. (2019). Toward CO2 utilization for direct power generation using an integrated system consisting of CO2 photoreduction with 3D TiO2/Ni-foam and a photocatalytic fuel cell. J. Mater. Chem. A 7, 6275–6284. doi:10.1039/c8ta12255e
Jin, Y., Luan, Y., Wu, Z., Wen, W., Zhang, X., and Wang, S. (2021). Photocatalytic fuel cell-assisted molecularly imprinted self-powered sensor: A flexible and sensitive tool for detecting aflatoxin B1. Anal. Chem. 93, 13204–13211. doi:10.1021/acs.analchem.1c02074
Kadam, S. R., Gosavi, S. W., Kale, B. B., Suzuki, N., Terashima, C., and Fujishima, A. (2019). Unique CdS@MoS2 core shell heterostructure for efficient hydrogen generation under natural sunlight. Sci. Rep. 9, 12036–12110. doi:10.1038/s41598-019-48532-3
Kaneko, M., Gokan, N., Katakura, N., Takei, Y., and Hoshino, M. (2005). Artificial photochemical nitrogen cycle to produce nitrogen and hydrogen from ammonia by platinized TiO2 and its application to a photofuel cell. Chem. Commun., 1625–1627. doi:10.1039/b418580c
Kee, M. W., Soo, J. W., Lam, S. M., Sin, J. C., and Mohamed, A. R. (2018). Evaluation of photocatalytic fuel cell (PFC) for electricity production and simultaneous degradation of methyl green in synthetic and real greywater effluents. J. Environ. Manage. 228, 383–392. doi:10.1016/j.jenvman.2018.09.038
Khalik, W. F., Ho, L. N., Ong, S. A., Voon, C. H., Wong, Y. S., Yusuf, S. Y., et al. (2018). Reactive Black 5 as electron donor and/or electron acceptor in dual chamber of solar photocatalytic fuel cell. Chemosphere 202, 467–475. doi:10.1016/j.chemosphere.2018.03.113
Khalik, W. F., Ong, S. A., Ho, L. N., Wong, Y. S., Voon, C. H., Yusuf, S. Y., et al. (2016). Influence of supporting electrolyte in electricity generation and degradation of organic pollutants in photocatalytic fuel cell. Environ. Sci. Pollut. Res. 23, 16716–16721. doi:10.1007/s11356-016-6840-9
Khalil, M., Naumi, F., Pratomo, U., Ivandini, T. A., Kadja, G. T. M., and Mulyana, J. Y. (2021). Coexposed TiO2’s (001) and (101) facets in TiO2/BiVO4 photoanodes for an enhanced photocatalytic fuel cell. Appl. Surf. Sci. 542, 148746. doi:10.1016/j.apsusc.2020.148746
Kim, W., Tachikawa, T., Monllor-Satoca, D., Kim, H., Majima, T., and Choi, W. (2013). Promoting water photooxidation on transparent WO3 thin films using an alumina overlayer. Energy Environ. Sci. 6, 3732. doi:10.1039/c3ee42151a
Lam, S.-M., Sin, J.-C., Lin, H., Li, H., Lim, J. W., and Zeng, H. (2020). A Z-scheme WO3 loaded-hexagonal rod-like ZnO/Zn photocatalytic fuel cell for chemical energy recuperation from food wastewater treatment. Appl. Surf. Sci. 514, 145945. doi:10.1016/j.apsusc.2020.145945
Lam, S. M., Ooi, J. K., Kee, M. W., and Sin, J. C. (2019). Photocatalytic fuel cell using TiO<sub>2</sub>/ZnO/Zn photoanode for greywater and bacteria abatements with power generation concomitantly. Key Eng. Mat. 821, 366–371. doi:10.4028/www.scientific.net/kem.821.366
Lee, S. L., Ho, L. N., Ong, S. A., Lee, G. M., Wong, Y. S., Voon, C. H., et al. (2016a). Comparative study of photocatalytic fuel cell for degradation of methylene blue under sunlight and ultra-violet light irradiation. Water Air Soil Pollut. 227, 445. doi:10.1007/s11270-016-3148-9
Lee, S. L., Ho, L. N., Ong, S. A., Wong, Y. S., Voon, C. H., Khalik, W. F., et al. (2017). A highly efficient immobilized ZnO/Zn photoanode for degradation of azo dye Reactive Green 19 in a photocatalytic fuel cell. Chemosphere 166, 118–125. doi:10.1016/j.chemosphere.2016.09.082
Lee, S. L., Ho, L. N., Ong, S. A., Wong, Y. S., Voon, C. H., Khalik, W. F., et al. (2016b). Enhanced electricity generation and degradation of the azo dye Reactive Green 19 in a photocatalytic fuel cell using ZnO/Zn as the photoanode. J. Clean. Prod. 127, 579–584. doi:10.1016/j.jclepro.2016.03.169
Lee, S. L., Ho, L. N., Ong, S. A., Wong, Y. S., Voon, C. H., Khalik, W. F., et al. (2018a). Exploring the relationship between molecular structure of dyes and light sources for photodegradation and electricity generation in photocatalytic fuel cell. Chemosphere 209, 935–943. doi:10.1016/j.chemosphere.2018.06.157
Lee, S. L., Ho, L. N., Ong, S. A., Wong, Y. S., Voon, C. H., Khalik, W. F., et al. (2018b). Role of dissolved oxygen on the degradation mechanism of Reactive Green 19 and electricity generation in photocatalytic fuel cell. Chemosphere 194, 675–681. doi:10.1016/j.chemosphere.2017.11.166
Leyland, N. S., Podporska-Carroll, J., Browne, J., Hinder, S. J., Quilty, B., and Pillai, S. C. (2016). Highly Efficient F, Cu doped TiO2 anti-bacterial visible light active photocatalytic coatings to combat hospital-acquired infections. Sci. Rep. 6, 24770–24810. doi:10.1038/srep24770
Li, J., Li, J., Chen, Q., Bai, J., and Zhou, B. (2013a). Converting hazardous organics into clean energy using a solar responsive dual photoelectrode photocatalytic fuel cell. J. Hazard. Mat. 262, 304–310. doi:10.1016/j.jhazmat.2013.08.066
Li, K., Xu, Y., He, Y., Yang, C., Wang, Y., and Jia, J. (2013b). Photocatalytic fuel cell (PFC) and dye self-photosensitization photocatalytic fuel cell (DSPFC) with BiOCl/Ti photoanode under UV and visible light irradiation. Environ. Sci. Technol. 47, 3490–3497. doi:10.1021/es303968n
Li, K., Zhang, H., Tang, T., Tang, Y., Wang, Y., and Jia, J. (2016a). Facile electrochemical polymerization of polypyrrole film applied as cathode material in dual rotating disk photo fuel cell. J. Power Sources 324, 368–377. doi:10.1016/j.jpowsour.2016.05.109
Li, L., Bai, J., Chen, S., Zhang, Y., Li, J., Zhou, T., et al. (2020). Enhanced O2− and HO via in situ generating H2O2 at activated graphite felt cathode for efficient photocatalytic fuel cell. Chem. Eng. J. 399, 125839. doi:10.1016/j.cej.2020.125839
Li, L., Li, J., Bai, J., Zeng, Q., Xia, L., Zhang, Y., et al. (2019a). The effect and mechanism of organic pollutants oxidation and chemical energy conversion for neutral wastewater via strengthening reactive oxygen species. Sci. Total Environ. 651, 1226–1235. doi:10.1016/j.scitotenv.2018.09.302
Li, L., Wang, G., Chen, R., Zhu, X., Wang, H., Liao, Q., et al. (2014). Optofluidics based micro-photocatalytic fuel cell for efficient wastewater treatment and electricity generation. Lab. Chip 14, 3368–3375. doi:10.1039/c4lc00595c
Li, M., Liu, Y., Dong, L., Shen, C., Li, F., Huang, M., et al. (2019b). Recent advances on photocatalytic fuel cell for environmental applications—the marriage of photocatalysis and fuel cells. Sci. Total Environ. 668, 966–978. doi:10.1016/j.scitotenv.2019.03.071
Li, N., Tang, S., Rao, Y., Qi, J., Wang, P., Jiang, Y., et al. (2018a). Improved dye removal and simultaneous electricity production in a photocatalytic fuel cell coupling with persulfate activation. Electrochim. Acta 270, 330–338. doi:10.1016/j.electacta.2018.03.083
Li, N., Tang, S., Rao, Y., Qi, J., Zhang, Q., and Yuan, D. (2019c). Peroxymonosulfate enhanced antibiotic removal and synchronous electricity generation in a photocatalytic fuel cell. Electrochim. Acta 298, 59–69. doi:10.1016/j.electacta.2018.12.063
Li, X., Liu, S., Cao, D., Mao, R., and Zhao, X. (2018b). Synergetic activation of H2O2 by photo-generated electrons and cathodic Fenton reaction for enhanced self-driven photoelectrocatalytic degradation of organic pollutants. Appl. Catal. B Environ. 235, 1–8. doi:10.1016/j.apcatb.2018.04.042
Li, X., Wang, G., Jing, L., Ni, W., Yan, H., Chen, C., et al. (2016b). A photoelectrochemical methanol fuel cell based on aligned TiO2 nanorods decorated graphene photoanode. Chem. Commun. 52, 2533–2536. doi:10.1039/c5cc09929c
Li, X. Z., and Li, F. B. (2001). Study of Au/Au3+-TiO2 photocatalysts toward visible photooxidation for water and wastewater treatment. Environ. Sci. Technol. 35, 2381–2387. doi:10.1021/es001752w
Liang, X., Liu, J., Zeng, D., Li, C., Chen, S., and Li, H. (2016). Hydrogen generation promoted by photocatalytic oxidation of ascorbate and glucose at a cadmium sulfide electrode. Electrochim. Acta 198, 40–48. doi:10.1016/j.electacta.2016.03.023
Lianos, P. (2011). Production of electricity and hydrogen by photocatalytic degradation of organic wastes in a photoelectrochemical cell. The concept of the photofuelcell: A review of a re-emerging research field. J. Hazard. Mat. 185, 575–590. doi:10.1016/j.jhazmat.2010.10.083
Lianos, P. (2017). Review of recent trends in photoelectrocatalytic conversion of solar energy to electricity and hydrogen. Appl. Catal. B Environ. 210, 235–254. doi:10.1016/j.apcatb.2017.03.067
Liao, Q., Li, L., Chen, R., Zhu, X., Wang, H., Ye, D., et al. (2015). Respective electrode potential characteristics of photocatalytic fuel cell with visible-light responsive photoanode and air-breathing cathode. Int. J. Hydrogen Energy 40, 16547–16555. doi:10.1016/j.ijhydene.2015.10.002
Liu, J., Xia, M., Chen, R., Zhu, X., Liao, Q., Ye, D., et al. (2019a). A membrane-less visible-light responsive micro photocatalytic fuel cell with the laterally-arranged CdS/ZnS-TiO2 photoanode and air-breathing CuO photocathode for simultaneous wastewater treatment and electricity generation. Sep. Purif. Technol. 229, 115821. doi:10.1016/j.seppur.2019.115821
Liu, X. H., Du, F., Chen, Q. Y., and Wang, Y. H. (2017). An effective self-driven PFC-PEC hybrid system for hydrogen generation from organic substance. Electrochim. Acta 245, 379–385. doi:10.1016/j.electacta.2017.05.163
Liu, X. H., Xing, Z. H., Chen, Q. Y., and Wang, Y. H. (2019b). Multi-functional photocatalytic fuel cell for simultaneous removal of organic pollutant and chromium (VI) accompanied with electricity production. Chemosphere 237, 124457. doi:10.1016/j.chemosphere.2019.124457
Liu, Y., Li, J., Zhou, B., Chen, H., Wang, Z., and Cai, W. (2011a). A TiO2-nanotube-array-based photocatalytic fuel cell using refractory organic compounds as substrates for electricity generation. Chem. Commun. 47, 10314–10316. doi:10.1039/c1cc13388h
Liu, Y., Li, J., Zhou, B., Li, X., Chen, H., Chen, Q., et al. (2011b). Efficient electricity production and simultaneously wastewater treatment via a high-performance photocatalytic fuel cell. Water Res. 45, 3991–3998. doi:10.1016/j.watres.2011.05.004
Liu, Y., Liu, L., and Yang, F. (2016). Energy-efficient degradation of rhodamine B in a LED illuminated photocatalytic fuel cell with anodic Ag/AgCl/GO and cathodic ZnIn2S4 catalysts. RSC Adv. 6, 12068–12075. doi:10.1039/c5ra25557k
Lu, Y., Chu, Y., Zheng, W., Huo, M., Huo, H., Qu, J., et al. (2019). Significant tetracycline hydrochloride degradation and electricity generation in a visible-light-driven dual photoelectrode photocatalytic fuel cell using BiVO4/TiO2 NT photoanode and Cu2O/TiO2 NT photocathode. Electrochim. Acta 320, 134617. doi:10.1016/j.electacta.2019.134617
Malato, S., Blaneo, J., Richter, C., and Miiow, B. (1999). Solar photocatalytic mineralization of commercial pesticides: Methamidophos. Chemosphere 38, 1145–1156. doi:10.1016/S0045-6535(98)00364-6
Mao, S. S., Shen, S., and Guo, L. (2012). Nanomaterials for renewable hydrogen production, storage and utilization. Prog. Nat. Sci. Mater. Int. 22, 522–534. doi:10.1016/j.pnsc.2012.12.003
Mariaswamy, A. J., Ong, S.-A., Abdul Rani, A. L., and Ho, L.-N. (2020). Comparison of different cathode materials for degradation of reactive red 120 and electricity generation in photocatalytic fuel cell. Water Air Soil Pollut. 231, 522. doi:10.1007/s11270-020-04870-x
Miseki, Y., and Sayama, K. (2019). Photocatalytic water splitting for solar hydrogen production using the carbonate effect and the Z-scheme reaction. Adv. Energy Mat. 9, 1801294–1801315. doi:10.1002/aenm.201801294
Mishra, P., Saravanan, P., Packirisamy, G., Jang, M., and Wang, C. (2021). A subtle review on the challenges of photocatalytic fuel cell for sustainable power production. Int. J. Hydrogen Energy 46, 22877–22906. doi:10.1016/j.ijhydene.2021.04.109
Morikawa, M., Ogura, Y., Ahmed, N., Kawamura, S., Mikami, G., Okamoto, S., et al. (2014). Photocatalytic conversion of carbon dioxide into methanol in reverse fuel cells with tungsten oxide and layered double hydroxide photocatalysts for solar fuel generation. Catal. Sci. Technol. 4, 1644–1651. doi:10.1039/c3cy00959a
Nahyoon, N. A., Liu, L., Rabé, K., Nahyoon, S. A., Abro, A. H., and Yang, F. (2019a). Efficient degradation of rhodamine B with sustainable electricity generation in a photocatalytic fuel cell using visible light Ag 3 PO 4/Fe/GTiP photoanode and ZnIn 2 S 4 photocathode. J. Taiwan Inst. Chem. Eng. 96, 137–147. doi:10.1016/j.jtice.2018.10.019
Nahyoon, N. A., Liu, L., Rabe, K., Thebo, K. H., Yuan, L., Sun, J., et al. (2018). Significant photocatalytic degradation and electricity generation in the photocatalytic fuel cell (PFC) using novel anodic nanocomposite of Fe, graphene oxide, and titanium phosphate. Electrochim. Acta 271, 41–48. doi:10.1016/j.electacta.2018.03.109
Nahyoon, N. A., Liu, L., Rabé, K., Yuan, L., Nahyoon, S. A., and Yang, F. (2019b). An ideal visible nanocomposite (Fe/GTiP) photoanode catalyst for treatment of antibiotics in water and simultaneous electricity generation in the photocatalytic fuel cell. Int. J. Hydrogen Energy 44, 21703–21715. doi:10.1016/j.ijhydene.2019.06.131
Nordin, N., Ho, L.-N., Ong, S.-A., Ibrahim, A. H., Abdul Rani, A. L., Lee, S.-L., et al. (2020). Hydroxyl radical formation in the hybrid system of photocatalytic fuel cell and peroxi-coagulation process affected by iron plate and UV light. Chemosphere 244, 125459. doi:10.1016/j.chemosphere.2019.125459
Nordin, N., Ho, L. N., Ong, S. A., Ibrahim, A. H., Lee, S. L., and Ong, Y. P. (2019). Elucidating the effects of different photoanode materials on electricity generation and dye degradation in a sustainable hybrid system of photocatalytic fuel cell and peroxi-coagulation process. Chemosphere 214, 614–622. doi:10.1016/j.chemosphere.2018.09.144
Nordin, N., Ho, L. N., Ong, S. A., Ibrahim, A. H., Wong, Y. S., Lee, S. L., et al. (2017). Hybrid system of photocatalytic fuel cell and Fenton process for electricity generation and degradation of Reactive Black 5. Sep. Purif. Technol. 177, 135–141. doi:10.1016/j.seppur.2016.12.030
Ogura, Y., Okamoto, S., Itoi, T., Fujishima, Y., Yoshida, Y., and Izumi, Y. (2014). A photofuel cell comprising titanium oxide and silver(I/0) photocatalysts for use of acidic water as a fuel. Chem. Commun. 50, 3067–3070. doi:10.1039/c4cc00194j
Ong, Y. P., Ho, L. N., Ong, S. A., Banjuraizah, J., Ibrahim, A. H., Lee, S. L., et al. (2019). A synergistic heterostructured ZnO/BaTiO3 loaded carbon photoanode in photocatalytic fuel cell for degradation of Reactive Red 120 and electricity generation. Chemosphere 219, 277–285. doi:10.1016/j.chemosphere.2018.12.004
O’Regan, B., and Grätzel, M. (1991). A low-cost, high-efficiency solar cell based on dye-sensitized colloidal TiO2 films. Nature 353, 737–740. doi:10.1038/353737a0
Pan, D., Xiao, S., Chen, X., Li, R., Cao, Y., Zhang, D., et al. (2019). Efficient photocatalytic fuel cell via simultaneous visible-photoelectrocatalytic degradation and electricity generation on a porous coral-like WO 3/W photoelectrode. Environ. Sci. Technol. 53, 3697–3706. doi:10.1021/acs.est.8b05685
Pan, H., Liao, W., Sun, N., Murugananthan, M., and Zhang, Y. (2018). Highly efficient and visible light responsive heterojunction composites as dual photoelectrodes for photocatalytic fuel cell. Catalysts 8, 30. doi:10.3390/catal8010030
Patsoura, A., Kondarides, D. I., and Verykios, X. E. (2007). Photocatalytic degradation of organic pollutants with simultaneous production of hydrogen. Catal. Today 124, 94–102. doi:10.1016/j.cattod.2007.03.028
Psaltis, D., Quake, S. R., and Yang, C. (2006). Developing optofluidic technology through the fusion of microfluidics and optics. Nature 442, 381–386. doi:10.1038/nature05060
Puga, A. V. (2016). Photocatalytic production of hydrogen from biomass-derived feedstocks. Coord. Chem. Rev. 315, 1–66. doi:10.1016/j.ccr.2015.12.009
Qian, B., Xu, Q., Wu, Y., Zhang, Y., Li, H., Wang, Y., et al. (2020). A highly efficient photocatalytic methanol fuel cell based on non-noble metal photoelectrodes: Study on its energy band engineering via experimental and density functional theory method. J. Power Sources 478, 228756. doi:10.1016/j.jpowsour.2020.228756
Qiu, M., Sun, P., Cui, G., Tong, Y., and Mai, W. (2019). A flexible microsupercapacitor with integral photocatalytic fuel cell for self-charging. ACS Nano 13, 8246–8255. doi:10.1021/acsnano.9b03603
Rabé, K., Liu, L., Nahyoon, N. A., Zhang, Y., and Idris, A. M. (2019a). Enhanced Rhodamine B and coking wastewater degradation and simultaneous electricity generation via anodic g-C 3 N 4/Fe 0 (1%)/TiO 2 and cathodic WO 3 in photocatalytic fuel cell system under visible light irradiation. Electrochim. Acta 298, 430–439. doi:10.1016/j.electacta.2018.12.121
Rabé, K., Liu, L., Nahyoon, N. A., Zhang, Y., and Idris, A. M. (2019b). Visible-light photocatalytic fuel cell with Z-scheme g-C3N4/Fe0/TiO2 anode and WO3 cathode efficiently degrades berberine chloride and stably generates electricity. Sep. Purif. Technol. 212, 774–782. doi:10.1016/j.seppur.2018.11.089
Raptis, D., Ploumistos, A., Zagoraiou, E., Thomou, E., Daletou, M., Sygellou, L., et al. (2018). Co-N doped reduced graphene oxide as oxygen reduction electrocatalyst applied to Photocatalytic Fuel Cells. Catal. Today 315, 31–35. doi:10.1016/j.cattod.2018.02.047
Rizzo, L., Meric, S., Guida, M., Kassinos, D., and Belgiorno, V. (2009). Heterogenous photocatalytic degradation kinetics and detoxification of an urban wastewater treatment plant effluent contaminated with pharmaceuticals. Water Res. 43, 4070–4078. doi:10.1016/j.watres.2009.06.046
Roberta, L. Z., and Wilson F, J. (2001). Photocatalytic decomposition of seawater-soluble crude oil fractions using high surface area colloid nanoparticles of TiO2. J. Photochem. Photobiol. A Chem. 5887, 1–8.
Saravanan, R., Gracia, F., and Stephen, A. (2017). Basic principles, mechanism, and challenges of photocatalysis. Springer, 19–40. doi:10.1007/978-3-319-62446-4_2
Seger, B., Lu, G. Q., and Wang, L. (2012). Electrical power and hydrogen production from a photo-fuel cell using formic acid and other single-carbon organics. J. Mat. Chem. 22, 10709–10715. doi:10.1039/c2jm16635f
Sfaelou, S., Antoniadou, M., Trakakis, G., Dracopoulos, V., Tasis, D., Parthenios, J., et al. (2012). Buckypaper as Pt-free cathode electrode in photoactivated fuel cells. Electrochim. Acta 80, 399–404. doi:10.1016/j.electacta.2012.07.046
Sfaelou, S., Pop, L. C., Monfort, O., Dracopoulos, V., and Lianos, P. (2016). Mesoporous WO3 photoanodes for hydrogen production by water splitting and PhotoFuelCell operation. Int. J. Hydrogen Energy 41, 5902–5907. doi:10.1016/j.ijhydene.2016.02.063
Sfaelou, S., Zhuang, X., Feng, X., and Lianos, P. (2015). Sulfur-doped porous carbon nanosheets as high performance electrocatalysts for PhotoFuelCells. RSC Adv. 5, 27953–27963. doi:10.1039/c5ra02027a
Shu, D., Wu, J., Gong, Y., Li, S., Hu, L., Yang, Y., et al. (2014). BiOI-based photoactivated fuel cell using refractory organic compounds as substrates to generate electricity. Catal. Today 224, 13–20. doi:10.1016/j.cattod.2013.12.017
Strataki, N., and Lianos, P. (2008). Optimization of parameters for hydrogen production by photocatalytic alcohol reforming in the presence of Pt/TiO2 nanocrystalline thin films. J. Adv. Oxid. Technol. 11, 111–115. doi:10.1515/jaots-2008-0114
Sui, M., Dong, Y., and You, H. (2015). Enhanced photocatalytic activity for the degradation of rhodamine B by integrating salinity gradient power into a photocatalytic fuel cell. RSC Adv. 5, 94184–94190. doi:10.1039/c5ra20093h
Sun, M., Zhu, Y., Yan, K., and Zhang, J. (2019). Dual-mode visible light-induced aptasensing platforms for bleomycin detection based on CdS–In2S3 heterojunction. Biosens. Bioelectron. X. 145, 111712. doi:10.1016/j.bios.2019.111712
Sun, Q., Han, B., Li, K., Yu, L., and Dong, L. (2022). The synergetic degradation of organic pollutants and removal of Cr(VI) in a multifunctional dual-chamber photocatalytic fuel cell with Ag@Fe2O3 cathode. Sep. Purif. Technol. 281, 119966. doi:10.1016/j.seppur.2021.119966
Tang, S., Li, N., Yuan, D., Tang, J., Li, X., Zhang, C., et al. (2019). Comparative study of persulfate oxidants promoted photocatalytic fuel cell performance: Simultaneous dye removal and electricity generation. Chemosphere 234, 658–667. doi:10.1016/j.chemosphere.2019.06.112
Tang, T., Li, K., Shen, Z., Sun, T., Wang, Y., and Jia, J. (2016). Facile synthesis of polypyrrole functionalized nickel foam with catalytic activity comparable to Pt for the poly-generation of hydrogen and electricity. J. Power Sources 301, 54–61. doi:10.1016/j.jpowsour.2015.09.126
Tang, T., Li, K., Ying, D., Sun, T., Wang, Y., and Jia, J. (2014). High efficient aqueous-film rotating disk photocatalytic fuel cell (RDPFC) with triple functions: Cogeneration of hydrogen and electricity with dye degradation. Int. J. Hydrogen Energy 39, 10258–10266. doi:10.1016/j.ijhydene.2014.04.134
Tedesco, M., Cipollina, A., Tamburini, A., Micale, G., Helsen, J., and Papapetrou, M. (2015). REAPower: Use of desalination brine for power production through reverse electrodialysis. Desalination Water Treat. 53, 3161–3169. doi:10.1080/19443994.2014.934102
Thor, S.-H., Ho, L.-N., Ong, S.-A., Nordin, N., Ong, Y.-P., and Yap, K.-L. (2020). Explicating the importance of aeration and pH for Amaranth degradation and electricity generation in a viable hybrid system of photocatalytic fuel cell and electro-Fenton process. Sep. Purif. Technol. 239, 116535. doi:10.1016/j.seppur.2020.116535
Ueno, H., Nemoto, J., Ohnuki, K., Horikawa, M., Hoshino, M., and Kaneko, M. (2009). Photoelectrochemical reaction of biomass-related compounds in a biophotochemical cell comprising a nanoporous TiO2 film photoanode and an O2-reducing cathode. J. Appl. Electrochem. 39, 1897–1905. doi:10.1007/s10800-009-9897-z
Vasseghian, Y., Khataee, A., Dragoi, E.-N., Moradi, M., Nabavifard, S., Oliveri Conti, G., et al. (2020). Pollutants degradation and power generation by photocatalytic fuel cells: A comprehensive review. Arab. J. Chem. 13, 8458–8480. doi:10.1016/j.arabjc.2020.07.016
Veerman, J., Saakes, M., Metz, S. J., and Harmsen, G. J. (2010). Electrical power from sea and river water by reverse electrodialysis: A first step from the laboratory to a real power plant. Environ. Sci. Technol. 44, 9207–9212. doi:10.1021/es1009345
Wang, B., Zhang, H., Lu, X. Y., Xuan, J., and Leung, M. K. H. (2014). Solar photocatalytic fuel cell using CdS-TiO2 photoanode and air-breathing cathode for wastewater treatment and simultaneous electricity production. Chem. Eng. J. 253, 174–182. doi:10.1016/j.cej.2014.05.041
Wang, H. N., Chen, X., Chen, R., Zhu, X., Liao, Q., Ye, D. D., et al. (2019). A ternary hybrid CuS/Cu2O/Cu nanowired photocathode for photocatalytic fuel cell. J. Power Sources 435, 226766. doi:10.1016/j.jpowsour.2019.226766
Wang, S., Li, Y., Wang, X., Zi, G., Zhou, C., Liu, B., et al. (2022a). One-step supramolecular preorganization constructed crinkly graphitic carbon nitride nanosheets with enhanced photocatalytic activity. J. Mat. Sci. Technol. 104, 155–162. doi:10.1016/j.jmst.2021.07.014
Wang, S., Liu, B., Wang, X., Zhang, Y., and Huang, W. (2022b). Nanoporous MoO3−x/BiVO4 photoanodes promoting charge separation for efficient photoelectrochemical water splitting. Nano Res. 15, 7026–7033. doi:10.1007/s12274-022-4344-0
Wang, S., Wang, L., and Huang, W. (2020a). Bismuth-based photocatalysts for solar energy conversion. J. Mat. Chem. A 8, 24307–24352. doi:10.1039/D0TA09729B
Wang, S., Wang, X., Liu, B., Guo, Z., Ostrikov, K., Ken), , et al. (2021a). Vacancy defect engineering of BiVO 4 photoanodes for photoelectrochemical water splitting. Nanoscale 13, 17989–18009. doi:10.1039/D1NR05691C
Wang, S., Wang, X., Liu, B., Xiao, X., Wang, L., and Huang, W. (2022c). Boosting the photocatalytic hydrogen production performance of graphitic carbon nitride nanosheets by tailoring the cyano groups. J. Colloid Interface Sci. 610, 495–503. doi:10.1016/j.jcis.2021.11.098
Wang, X., Liu, B., Xiao, X., Wang, S., and Huang, W. (2021b). Boron dopant simultaneously achieving nanostructure control and electronic structure tuning of graphitic carbon nitride with enhanced photocatalytic activity. J. Mat. Chem. C 9, 14876–14884. doi:10.1039/D1TC04142H
Wang, Y., Lan, Y., Bu, D., Qian, B., Wang, Y., Wang, B., et al. (2020b). A study on tandem photoanode and photocathode for photocatalytic formaldehyde fuel cell. Electrochim. Acta 352, 136476. doi:10.1016/j.electacta.2020.136476
Wang, Y., Shang, X., Shen, J., Zhang, Z., Wang, D., Lin, J., et al. (2020c). Direct and indirect Z-scheme heterostructure-coupled photosystem enabling cooperation of CO2 reduction and H2O oxidation. Nat. Commun. 11, 3043–3111. doi:10.1038/s41467-020-16742-3
Wang, Y., Wang, Y., Song, X., Zhang, Y., and Ma, T. (2020d). BiOCl-based photocathode for photocatalytic fuel cell. Appl. Surf. Sci. 506, 144949. doi:10.1016/j.apsusc.2019.144949
Wu, Z., Zhao, G., Zhang, Y., Liu, J., Zhang, Y. N., and Shi, H. (2015). A solar-driven photocatalytic fuel cell with dual photoelectrode for simultaneous wastewater treatment and hydrogen production. J. Mat. Chem. A 3, 3416–3424. doi:10.1039/c4ta06604a
Xia, L., Bai, J., Li, J., Li, L., Chen, S., Xu, Q., et al. (2018). High-efficient energy recovery from organics degradation for neutral wastewater treatment based on radicals catalytic reaction of Fe2+/Fe3+-EDTA complexes. Chemosphere 201, 59–65. doi:10.1016/j.chemosphere.2018.02.150
Xia, L., Bai, J., Li, J., Zeng, Q., Li, X., and Zhou, B. (2016a). A highly efficient BiVO4/WO3/W heterojunction photoanode for visible-light responsive dual photoelectrode photocatalytic fuel cell. Appl. Catal. B Environ. 183, 224–230. doi:10.1016/j.apcatb.2015.10.050
Xia, M., Chen, R., Zhu, X., Liao, Q., An, L., Wang, Z., et al. (2016b). A micro photocatalytic fuel cell with an air-breathing, membraneless and monolithic design. Sci. Bull. (Beijing). 61, 1699–1710. doi:10.1007/s11434-016-1178-8
Xiao, K., He, J., Zhang, J., Yang, B., and Zhao, X. (2019). Simultaneous Cr(VI) removal and bisphenol A degradation in a solar-driven photocatalytic fuel cell with dopamine modified carbon felt cathode. Appl. Surf. Sci. 471, 912–920. doi:10.1016/j.apsusc.2018.12.079
Xie, F., Chen, R., Zhu, X., Liao, Q., Ye, D., Zhang, B., et al. (2019). CO2 utilization: Direct power generation by a coupled system that integrates photocatalytic reduction of CO2 with photocatalytic fuel cell. J. CO2 Util. 32, 31–36. doi:10.1016/j.jcou.2019.03.017
Xie, S., and Ouyang, K. (2017). Degradation of refractory organic compounds by photocatalytic fuel cell with solar responsive WO3/FTO photoanode and air-breathing cathode. J. Colloid Interface Sci. 500, 220–227. doi:10.1016/j.jcis.2017.04.002
Xie, S., Ouyang, K., and Shao, Y. (2017). A solar responsive photocatalytic fuel cell with a heterostructured ZnFe2O4/TiO2-NTs photoanode and an air-breathing cathode. Int. J. Hydrogen Energy 42, 29201–29209. doi:10.1016/j.ijhydene.2017.10.059
Xie, S., Ouyang, K., and Ye, X. (2018). A novel visible-light responsive photocatalytic fuel cell with a heterostructured BiVO4/WO3 photoanode and a Pt/C air-breathing cathode. J. Colloid Interface Sci. 532, 758–766. doi:10.1016/j.jcis.2018.07.032
Xu, P., and Xu, H. (2019). Enhanced electricity generation and H 2 O 2 production in a photocatalytic fuel cell and fenton hybrid system Assisted with reverse electrodialysis. ACS Omega 4, 5848–5851. doi:10.1021/acsomega.9b00282
Xu, P., Xu, H., Zheng, D., Ma, J., and Hou, B. (2019b). The reverse electrodialysis driven electrochemical process assisted by anodic photocatalysis for hydrogen peroxide production. Chemosphere 237, 124509. doi:10.1016/j.chemosphere.2019.124509
Xu, P., Xu, H., and Zheng, D. (2019a). Simultaneous electricity generation and wastewater treatment in a photocatalytic fuel cell integrating electro-Fenton process. J. Power Sources 421, 156–161. doi:10.1016/j.jpowsour.2019.03.033
Xu, P., Zheng, D., and Xu, H. (2019c). The feasibility and mechanism of reverse electrodialysis enhanced photocatalytic fuel cell-Fenton system on advanced treatment of coal gasification wastewater. Sep. Purif. Technol. 220, 183–188. doi:10.1016/j.seppur.2019.03.064
Xu, Y., He, Y., Cao, X., Zhong, D., and Jia, J. (2008). TiO2/Ti rotating disk photoelectrocatalytic (PEC) reactor: A combination of highly effective thin-film PEC and conventional PEC processes on a single electrode. Environ. Sci. Technol. 42, 2612–2617. doi:10.1021/es702921h
Xu, Y., He, Y., Jia, J., Zhong, D., and Wang, Y. (2009). Cu-TiO2/Ti dual rotating disk photocatalytic (PC) reactor: Dual electrode degradation facilitated by spontaneous electron transfer. Environ. Sci. Technol. 43, 6289–6294. doi:10.1021/es901269s
Yan, K., Ji, W., Zhu, Y., Chen, F., and Zhang, J. (2019). Photofuel cell coupling with redox cycling as a highly sensitive and selective self-powered sensing platform for the detection of tyrosinase activity. Chem. Commun. 55, 12040–12043. doi:10.1039/c9cc05649a
Yan, K., Yang, Y., Okoth, O. K., Cheng, L., and Zhang, J. (2016). Visible-light induced self-powered sensing platform based on a photofuel cell. Anal. Chem. 88, 6140–6144. doi:10.1021/acs.analchem.6b01600
Yan, K., Yang, Y., and Zhang, J. (2018a). A self-powered sensor based on molecularly imprinted polymer-coupled graphitic carbon nitride photoanode for selective detection of bisphenol A. Sensors Actuators B Chem. 259, 394–401. doi:10.1016/j.snb.2017.12.075
Yan, K., Yang, Y., Zhu, Y., and Zhang, J. (2017). Highly selective self-powered sensing platform for p-nitrophenol detection constructed with a photocathode-based photocatalytic fuel cell. Anal. Chem. 89, 8599–8603. doi:10.1021/acs.analchem.7b02402
Yan, K., Zhu, Y., Ji, W., Chen, F., and Zhang, J. (2018b). Visible light-driven membraneless photocatalytic fuel cell toward self-powered aptasensing of PCB77. Anal. Chem. 90, 9662–9666. doi:10.1021/acs.analchem.8b02302
Yang, J., Liao, W., Liu, Y., Murugananthan, M., and Zhang, Y. (2014). Degradation of rhodamine B using a visible-light driven photocatalytic fuel cell. Electrochim. Acta 144, 7–15. doi:10.1016/j.electacta.2014.08.036
Yang, Y., Yan, K., and Zhang, J. (2017). Dual non-enzymatic glucose sensing on Ni(OH)2/TiO2 photoanode under visible light illumination. Electrochim. Acta 228, 28–35. doi:10.1016/j.electacta.2017.01.050
Yao, X., Gao, J., Yan, K., Chen, Y., and Zhang, J. (2020). Ratiometric self-powered sensor for 17β-estradiol detection based on a dual-channel photocatalytic fuel cell. Anal. Chem. 92, 8026–8030. doi:10.1021/acs.analchem.0c01543
Ye, Y., Bruning, H., Li, X., Yntema, D., and Rijnaarts, H. H. M. (2018). Significant enhancement of micropollutant photocatalytic degradation using a TiO2 nanotube array photoanode based photocatalytic fuel cell. Chem. Eng. J. 354, 553–562. doi:10.1016/j.cej.2018.08.064
Ying, D., Cao, R., Li, C., Tang, T., Li, K., Wang, H., et al. (2016). Study of the photocurrent in a photocatalytic fuel cell for wastewater treatment and the effects of TiO2 surface morphology to the apportionment of the photocurrent. Electrochim. Acta 192, 319–327. doi:10.1016/j.electacta.2016.01.210
Yu, H., Xue, Y., Lu, Y., Wang, X., Zhu, S., Qin, W., et al. (2020). Novel application of a Z-scheme photocatalyst of Ag3PO4@g-C3N4 for photocatalytic fuel cells. J. Environ. Manage. 254, 109738. doi:10.1016/j.jenvman.2019.109738
Yu, Y., Xie, F., Chen, R., Zhu, X., Liao, Q., Ye, D., et al. (2021). New insights into the role of CO2 in a photocatalytic fuel cell. J. Power Sources 487, 229438. doi:10.1016/j.jpowsour.2020.229438
Zeng, Q., Bai, J., Li, J., Li, L., Xia, L., Zhou, B., et al. (2018a). Highly-stable and efficient photocatalytic fuel cell based on an epitaxial TiO2/WO3/W nanothorn photoanode and enhanced radical reactions for simultaneous electricity production and wastewater treatment. Appl. Energy 220, 127–137. doi:10.1016/j.apenergy.2018.03.042
Zeng, Q., Chang, S., Beyhaqi, A., Lian, S., Xu, H., Xie, J., et al. (2020). Efficient solar hydrogen production coupled with organics degradation by a hybrid tandem photocatalytic fuel cell using a silicon-doped TiO2 nanorod array with enhanced electronic properties. J. Hazard. Mat. 394, 121425. doi:10.1016/j.jhazmat.2019.121425
Zeng, Q., Lyu, L., Gao, Y., Chang, S., and Hu, C. (2018b). A self-sustaining monolithic photoelectrocatalytic/photovoltaic system based on a WO3/BiVO4 photoanode and Si PVC for efficiently producing clean energy from refractory organics degradation. Appl. Catal. B Environ. 238, 309–317. doi:10.1016/j.apcatb.2018.07.005
Zhang, B., Fan, W., Yao, T., Liao, S., Li, A., Li, D., et al. (2017a). Design and fabrication of a dual-photoelectrode fuel cell towards cost-effective electricity production from biomass. ChemSusChem 10, 99–105. doi:10.1002/cssc.201601422
Zhang, B., Shi, J., Ding, C., Chong, R., Zhang, B., Wang, Z., et al. (2015a). Conversion of biomass derivatives to electricity in photo fuel cells using undoped and tungsten-doped bismuth vanadate photoanodes. ChemSusChem 8, 4049–4055. doi:10.1002/cssc.201500942
Zhang, B., Shi, J., Ding, C., Chong, R., Zhang, B., Wang, Z., et al. (2015b). Conversion of biomass derivatives to electricity in photo fuel cells using undoped and tungsten-doped bismuth vanadate photoanodes. ChemSusChem 8, 4049–4055. doi:10.1002/cssc.201500942
Zhang, B., Zhang, Q., He, L., Xia, Y., Meng, F., Liu, G., et al. (2019a). Photoelectrochemical oxidation of glucose on tungsten trioxide electrode for non-enzymatic glucose sensing and fuel cell applications. J. Electrochem. Soc. 166, B569–B575. doi:10.1149/2.0221908jes
Zhang, D., Wang, Y., Wang, Y., Zhang, Y., and Song, X.-M. (2020a). Fe2O3/NiO photocathode for photocatalytic methanol fuel cell: An insight on solar energy conversion. J. Alloys Compd. 815, 152377. doi:10.1016/j.jallcom.2019.152377
Zhang, J., Li, L., Zheng, J., Yang, P., Wu, X., Cheng, C., et al. (2019b). Improved organic pollutants removal and simultaneous electricity production via integrating Fenton process and dual rotating disk photocatalytic fuel cell system using bamboo charcoal cathode. Chem. Eng. J. 361, 1198–1206. doi:10.1016/j.cej.2018.12.178
Zhang, J., Lv, S., Zheng, J., Yang, P., Li, J., Wu, X., et al. (2020b). Self-CO 2 recycling photocatalytic fuel cell for enhancing degradation of pollutants and production of carbon-neutral fuel. ACS Sustain. Chem. Eng. 8, 11133–11140. doi:10.1021/acssuschemeng.0c01906
Zhang, J., Yang, P., Zheng, J., Li, J., Fu, Q., He, Y., et al. (2020c). Enhanced carbon dioxide reduction in a thin-film rotating disk photocatalytic fuel cell reactor. J. CO2 Util. 37, 328–334. doi:10.1016/j.jcou.2020.01.006
Zhang, J., Yang, P., Zheng, J., Li, J., Lv, S., Jin, T., et al. (2020d). Degradation of gaseous HCHO in a rotating photocatalytic fuel cell system with an absorption efficiency of up to 94%. Chem. Eng. J. 392, 123634. doi:10.1016/j.cej.2019.123634
Zhang, J., Yang, P., Zheng, J., Li, L., Wu, X., Cheng, C., et al. (2019c). Multi-cathode photocatalytic fuel cell with rotating bamboo charcoal electrodes for electricity production and simultaneous organic pollutants removal. Int. J. Hydrogen Energy 44, 28803–28811. doi:10.1016/j.ijhydene.2019.08.262
Zhang, J., Zheng, J., and Yang, W. (2021). Green supercapacitor assisted photocatalytic fuel cell system for sustainable hydrogen production. Chem. Eng. J. 403, 126368. doi:10.1016/j.cej.2020.126368
Zhang, L., Liang, C., Guo, H., Niu, C. G., Zhao, X. F., Wen, X. J., et al. (2019d). Construction of a high-performance photocatalytic fuel cell (PFC) based on plasmonic silver modified Cr-BiOCl nanosheets for simultaneous electricity production and pollutant removal. Nanoscale 11, 6662–6676. doi:10.1039/c8nr09616c
Zhang, L., Niu, C. G., Xie, G. X., Wen, X. J., Zhang, X. G., and Zeng, G. M. (2017b). Controlled growth of BiOCl with large {010} facets for dye self-photosensitization photocatalytic fuel cells application. ACS Sustain. Chem. Eng. 5, 4619–4629. doi:10.1021/acssuschemeng.6b03150
Zhang, L., Niu, C. G., Zhao, X. F., Liang, C., Guo, H., and Zeng, G. M. (2018a). Ultrathin BiOCl single-crystalline nanosheets with large reactive facets area and high electron mobility efficiency: A superior candidate for high-performance dye self-photosensitization photocatalytic fuel cell. ACS Appl. Mat. Interfaces 10, 39723–39734. doi:10.1021/acsami.8b14227
Zhang, Y., Li, J., Bai, J., Li, X., Shen, Z., Xia, L., et al. (2018b). Total organic carbon and total nitrogen removal and simultaneous electricity generation for nitrogen-containing wastewater based on the catalytic reactions of hydroxyl and chlorine radicals. Appl. Catal. B Environ. 238, 168–176. doi:10.1016/j.apcatb.2018.07.036
Zhang, Y., Liu, L., Chen, Q., He, Y., and Leung, M. K. H. (2019e). Electricity generating & high efficiency advanced oxidation process including peroxymonosulfate activation in photocatalytic fuel cell. Chem. Eng. J. 378, 122148. doi:10.1016/j.cej.2019.122148
Zhao, K., Zeng, Q., Bai, J., Li, J., Xia, L., Chen, S., et al. (2017). Enhanced organic pollutants degradation and electricity production simultaneously via strengthening the radicals reaction in a novel Fenton-photocatalytic fuel cell system. Water Res. 108, 293–300. doi:10.1016/j.watres.2016.11.002
Zhao, Z., Tian, J., Sang, Y., Cabot, A., and Liu, H. (2015). Structure, synthesis, and applications of TiO2 nanobelts. Adv. Mat. 27, 2557–2582. doi:10.1002/adma.201405589
Glossary
AMSC— asymmetric micro super capacitor
AEM— anode exchange membrane
BJS— buried iunction silicon
BPA— bisphenol A
BNC— biomass derived N-doped carbon
CEM— cathode exchange membrane
DCSP— dual chamber single photoelectrode
EF— electro-fenton
HC— high concentration
LDH— layered double halides
LC— low concentration
MIP— molecularly imprinted polymer
MPD— maximum power density
MFC— micro fuel cell
OCV— open circuit voltage
ORR— oxygen reduction reaction
PEM— proton exchange membrane
PVC— photovoltaic cell
PFC— photocatalytic fuel cell
PEC— photoelectrochemical cell
RDPFC— rotating disc photocatalytic fuel cell
Rh B— rhodamine B
RED— reverse electro dialysis
SCSP— single chamber single photoelectrode
SCDP— single chamber dual photoelectrode
SGP— salinity gradient power
SMPP— self-sustaining monolithic photocatalytic/photovoltaic system
SCC— short circuit current
STNR— silicon doped TiO2 nano rod array
SSC— silicon solar cell
TOC— total organic carbon
Keywords: photocatalytic fuel cells, hydrogen evolution (HE), CO2 reduction, heavy metal reduction, sensor, photocatalysis
Citation: John S, Nogala W, Gupta B and Singh S (2022) Synergy of photocatalysis and fuel cells: A chronological review on efficient designs, potential materials and emerging applications. Front. Chem. 10:1038221. doi: 10.3389/fchem.2022.1038221
Received: 06 September 2022; Accepted: 10 November 2022;
Published: 25 November 2022.
Edited by:
Rama Krishna Chava, Yeungnam University, South KoreaReviewed by:
Rajesh Tayade, Central Salt and Marine Chemicals Research Institute (CSIR), IndiaSongcan Wang, Northwestern Polytechnical University, China
Gouse Peera Shaik, Keimyung University, South Korea
Copyright © 2022 John, Nogala, Gupta and Singh. This is an open-access article distributed under the terms of the Creative Commons Attribution License (CC BY). The use, distribution or reproduction in other forums is permitted, provided the original author(s) and the copyright owner(s) are credited and that the original publication in this journal is cited, in accordance with accepted academic practice. No use, distribution or reproduction is permitted which does not comply with these terms.
*Correspondence: Bhavana Gupta, bgupta@ichf.edu.pl; Shubra Singh, shubra6@gmail.com